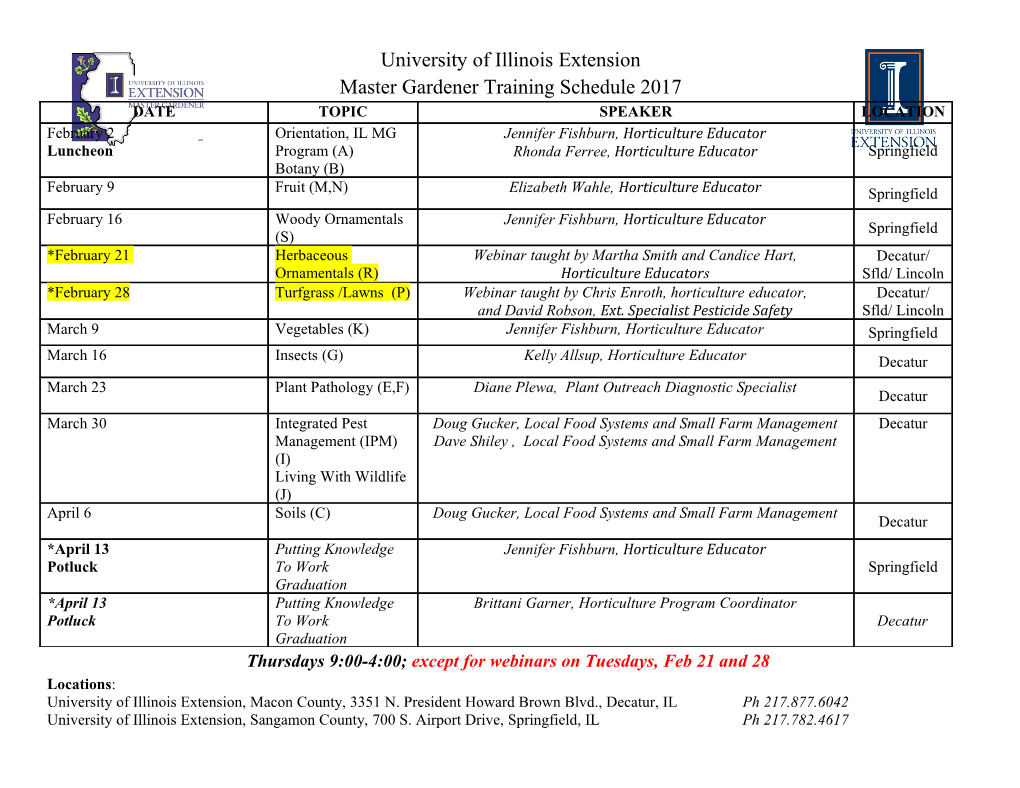
Publications 10-25-2017 Constraining Balmer Alpha Fine Structure Excitation Measured in Geocoronal Hydrogen Observations D. D. Gardner University of Wisconsin-Madison E. J. Mierkiewicz Embry-Riddle Aeronautical University, [email protected] F. L. Roesler University of Wisconsin-Madison S. M. Nossal University of Wisconsin-Madison L. M. Haffner University of Wisconsin-Madison Follow this and additional works at: https://commons.erau.edu/publication Part of the Astrophysics and Astronomy Commons Scholarly Commons Citation Gardner, D. D., Mierkiewicz, E. J., Roesler, F. L., Nossal, S. M., & Haffner, L. M. (2017). Constraining Balmer Alpha Fine Structure Excitation Measured in Geocoronal Hydrogen Observations. Journal of Geophysical Research: Space Physics, 122(10). https://doi.org/10.1002/2017JA024055 This Article is brought to you for free and open access by Scholarly Commons. It has been accepted for inclusion in Publications by an authorized administrator of Scholarly Commons. For more information, please contact [email protected]. Journal of Geophysical Research: Space Physics RESEARCH ARTICLE Constraining Balmer Alpha Fine Structure Excitation Measured 10.1002/2017JA024055 in Geocoronal Hydrogen Observations Key Points: • The Balmer beta/alpha line ratio is D. D. Gardner1 , E. J. Mierkiewicz2 , F. L. Roesler1, S. M. Nossal1 , and L. M. Haffner1 explicitly shown to be a function 1 2 of viewing geometry and solar University of Wisconsin-Madison, Madison, Wisconsin, USA, Embry-Riddle Aeronautical University-Daytona, conditions Daytona Beach, Florida, USA • Cascade determined directly from Balmer alpha spectral fits corroborates Balmer beta/alpha line Abstract Cascade contributions to geocoronal Balmer airglow line profiles are directly proportional ratio behavior • The Balmer ∕ line ratio constrains to the Balmer ∕ line ratio and can therefore be determined with near simultaneous Balmer forward modeled [H(z)] distributions observations. Due to scattering differences for solar Lyman and Lyman (responsible for the terrestrial and Balmer alpha line profile Balmer and Balmer fluorescence, respectively), there is an expected trend for the cascade emission to kinematics become a smaller fraction of the Balmer intensity at larger shadow altitudes. Near-coincident Balmer and Balmer data sets, obtained from the Wisconsin H alpha Mapper Fabry-Perot, are used to determine the Correspondence to: cascade contribution to the Balmer line profile and to show, for the first time, the Balmer line ratio, as D. Gardner, ∕ [email protected] a function of shadow altitude. We show that this result is in agreement with direct cascade determinations from Balmer line profile fits obtained independently by high-resolution Fabry-Perot at Pine Bluff, WI. We also demonstrate with radiative transport forward modeling that a solar cycle influence on cascade is Citation: Gardner, D. D., Mierkiewicz, E. J., expected, and that the Balmer ∕ line ratio poses a tight constraint on retrieved aeronomical parameters Roesler, F. L., Nossal, S. M., & (such as hydrogen’s evaporative escape rate and exobase density). Haffner, L. M. (2017). Constraining Balmer alpha fine structure Understanding how hydrogen densities and temperatures change at excitation measured in geocoronal Plain Language Summary hydrogen observations. Journal the top of the atmosphere (near space, actually) is important for climate change and atmospheric evolution of Geophysical Research: Space investigations. This paper shows how the density distribution of hydrogen at the top of the atmosphere Physics, 122, 10,727–10,747. can be inferred using ground-based observations of hydrogen airglow emissions and modeling. The unique https://doi.org/10.1002/2017JA024055 variation of these airglow emissions is a useful constraint to help better determine neutral hydrogen temperatures and densities at the top of the atmosphere. Received 19 FEB 2017 Accepted 15 SEP 2017 Accepted article online 19 SEP 2017 Published online 25 OCT 2017 1. Introduction Ground-based geocoronal hydrogen Balmer airglow measurements provide a unique opportunity to con- tribute to three major areas of geocoronal research: geocoronal physics, atmospheric structure and coupling, and exospheric variability. Additionally, an important reason to study planetary exospheres is to learn about the evolutionary loss of species through various escape mechanisms (Hunten & Donahue, 1976). Toward this end, measurements of geocoronal hydrogen, H, enable a better understanding of the physical pro- cesses underlying the abundance, transport, and variability of hydrogen through Earth’s upper atmosphere (Mierkiewicz et al., 1999). Hydrogen is the dominant and lightest species in the near-collisionless exosphere, and the daughter product of H2O, H2, and CH4 photolysis in the well-mixed mesosphere. Accurately quanti- fying hydrogen’s vertical density distribution profile, [H(z)], photochemically induced vertical transport flux, , and related exospheric dynamics is a challenging problem critical to not only the study of H aeronomy but also a wide range of atmospheric, near space, and climatological investigations, as well as their associated model validations (Fahr & Shizgal, 1983; Pierrehumbert, 2010). For example, magnetospheric energy dissi- pation through ring current decay (Daglis et al., 1999), associated plasmaspheric refilling rates (Krall, Huba, et al., 2016; Krall, Emmert, et al., 2016; Krall & Huba, 2016), and the geocoronal response itself to geomagnetic activity (Zoenchen et al., 2017) all depend on the underlying H distribution. The hydrogen distribution in the upper atmosphere can be constrained by forward model analysis of remote sensing airglow data obtained by rocket, satellite, and/or ground-based observations. Radiative transport code can be coupled to appropriate atmospheric models, and the observed hydrogen airglow phenomena can be replicated by varying the model input parameters (Bishop et al., 2001, 2004). In one such approach, ©2017. American Geophysical Union. multiple observations along distinct lines of sight, sampling a wide range of viewing geometries, can be All Rights Reserved. used to parameterize forward model retrievals of geocoronal hydrogen parameters (Anderson et al., 1987). GARDNER ET AL. CONSTRAINING H ALPHA FINE STRUCTURE IN GEOCORONAL HYDROGEN OBSERVATION 10,727 Journal of Geophysical Research: Space Physics 10.1002/2017JA024055 The temporal resolution of these ground-based model/data retrieved aeronomical parameters depends on how quickly the observer can sample a sufficient range of viewing geometries to fully constrain the model. Thus, ground-based observations of the faint hydrogen airglow have typically been obtained by Fabry-Perot interferometers, favored because of their ability to work at relatively high resolving power and high through- put (Roesler, 1974). These instrumental characteristics are needed to accurately separate the airglow emission from the ubiquitous Galactic background while still being able to temporally determine and isolate source intensity variations with viewing geometry (Mierkiewicz et al., 2006). With regard to geocoronal physics, the interpretation of the Balmer line profile for kinematic signatures has been limited by uncertainties from excitation mechanisms beyond direct Lyman excitation such as cascade-induced emission and uncertainties due to residual spectral contamination in the line profile wings from Galactic and terrestrial sources. This, in part, has been due to difficulties in obtaining high spectral resolu- tion Balmer line profiles with the signal-to-noise ratio (SNR) required to explore perturbations of the Balmer profile at the 5 to 10% level. Toward this end, Roesler et al. (2014) established that simultaneous observa- tions of geocoronal Balmer and Balmer airglow intensities can be used to obtain the cascade contribution to the total Balmer line profile intensity; that is, the cascade is directly proportional to the Balmer ∕ line ratio. Accounting for the cascade contribution to the geocoronal Balmer spectral line shape is critical to prop- erly fit and interpret additional excitation mechanisms and exospheric dynamic signatures. For example, a non-thermalized hot hydrogen population in the upper thermosphere, as proposed by Qin and Waldrop (2016), could potentially be evident in sufficiently sensitive, low shadow altitude Balmer spectra as a broad, symmetric enhancement at the base of the emission line. In a second example, escaping populations of hydro- gen in deep shadow altitude observations are predicted to give rise to a faint red shifted spectral signature near the dominant fine structure cascade contribution to the Balmer line shape (see Chamberlain & Hunten, 1987; Yelle & Roesler, 1985). And while nonauroral collisional excitation components to Balmer have yet to be identified, photoelectron impact excitation may enhance fine structure components in Balmer in addi- tion to those from direct solar Lyman series excitation. Note, the expected photoelectron impact excitation contribution to Balmer is less than a single Rayleigh (Bishop et al., 2001); the Wisconsin H alpha Mapper instrument is sensitive to sub-Rayleigh sources. Building on the work of Roesler et al. (2014), here we use near-coincident ground-based observations of geo- coronal Balmer and Balmer line ratios, obtained near solar minimum in 2008 by the Wisconsin H alpha Mapper (WHAM), to corroborate cascade observed directly in high-resolution ground-based Balmer data sets obtained near solar maximum
Details
-
File Typepdf
-
Upload Time-
-
Content LanguagesEnglish
-
Upload UserAnonymous/Not logged-in
-
File Pages22 Page
-
File Size-