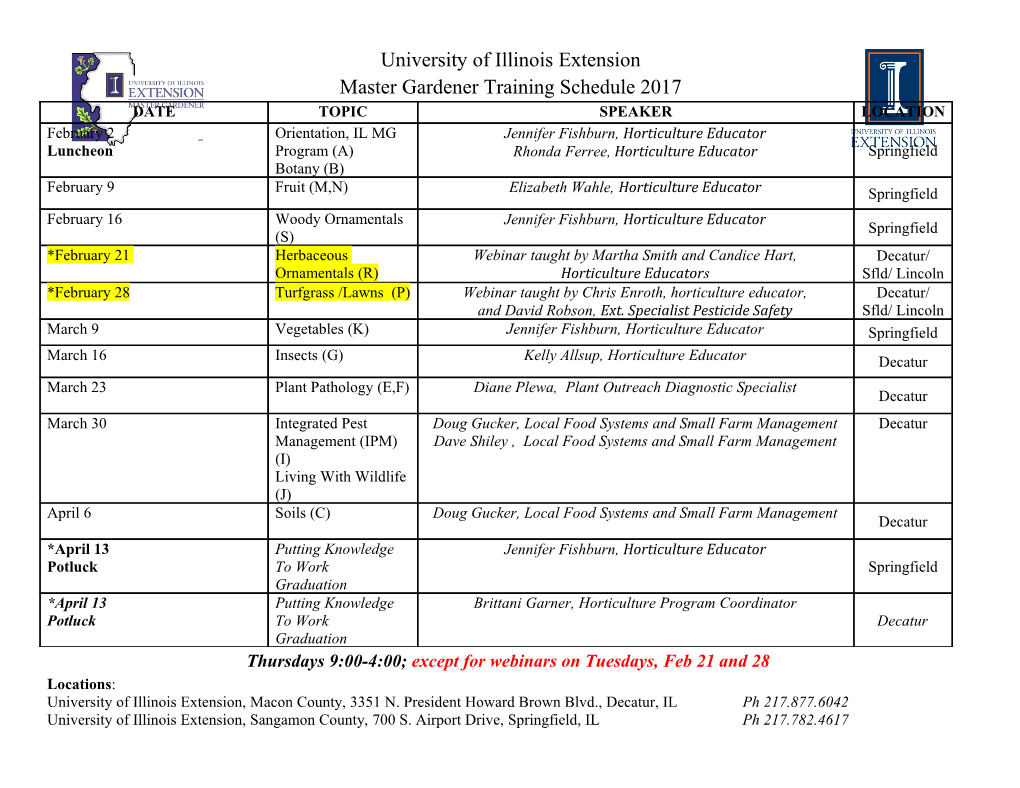
www.nature.com/scientificreports OPEN Exploring the Ligand Efcacy of Cannabinoid Receptor 1 (CB1) using Molecular Dynamics Simulations Received: 13 February 2018 Sang Won Jung 1, Art E. Cho2 & Wookyung Yu1,3,4 Accepted: 23 August 2018 Cannabinoid receptor 1 (CB1) is a promising therapeutic target for a variety of disorders. Distinct Published: xx xx xxxx efcacy profles showed diferent therapeutic efects on CB1 dependent on three classes of ligands: agonists, antagonists, and inverse agonists. To discriminate the distinct efcacy profles of the ligands, we carried out molecular dynamics (MD) simulations to identify the dynamic behaviors of inactive and active conformations of CB1 structures with the ligands. In addition, the molecular mechanics Poisson-Boltzmann surface area (MM-PBSA) method was applied to analyze the binding free energy decompositions of the CB1-ligand complexes. With these two methods, we found the possibility that the three classes of ligands can be discriminated. Our fndings shed light on the understanding of diferent efcacy profles of ligands by analyzing the structural behaviors of intact CB1 structures and the binding energies of ligands, thereby yielding insights that are useful for the design of new potent CB1 drugs. Cannabinoid receptors are class A members of the G-protein coupled receptor (GPCR) superfamily1. Two sub- types of cannabinoid receptors are currently known: cannabinoid receptor 1 (CB1)2,3, which is located in the brain and many peripheral organs and tissues, and cannabinoid receptor 2 (CB2)4, which is mainly expressed in immune cells. CB1 is the most abundant GPCR in the brain and central nervous system that regulates a variety of brain functions and behaviors such as pain, control of movement, memory, and neuroendocrine regulation5–7. In addition, CB1 in peripheral organs and tissues has been shown to play an important role in physiological mechanisms such as energy metabolism, appetite control, endocrine, and metabolic regulation8,9. Tus, CB1 is a promising therapeutic target for a variety of disorders. Depending on the biological response of CB1, ligands with different efficacy profiles and therapeutic efects were largely classifed into three classes: agonists, antagonists, and inverse agonists10–12. CB1 agonists have potential for therapeutic applications in pain, infammation, and neurodegenerative disorders13,14. Te Δ9-tetrahydrocannabinol (THC)15, which is the psychoactive constituent of marijuana, is known as a CB1 par- tial agonist and is used for therapeutic purposes such as analgesic, antiemetic, and anticonvulsant in the USA and other countries16,17. Meanwhile, CB1 antagonists and inverse agonists have been developed for therapeutic applications in obesity-related metabolic disorders, mental illness, liver fbrosis, and nicotine addiction18–20. Te Δ9-tetrahydrocannabivarin (THCV)21 is a CB1 antagonist that is structurally similar to THC. However, unlike THC, THCV has anti-obesity activity. CB1 inverse agonists such as rimonabant22 and taranabant23 are also efec- tive in treatment of obesity, but psychiatric side efects such as anxiety and depression have been reported24,25. Terefore, with regard to the distinct efcacy of the three classes of ligands, sophisticated drug design strategies are required to achieve the desired therapeutic efects. Recently, two conformations of CB1 crystal structures have been determined: (1) the inactive conformation bound to the antagonist AM653826 or inverse agonist taranabant27; and (2) the active conformation bound to the agonist AM11542 or AM84128. Tere were signifcant structural changes between the two conformations, especially in helices I, II, and VI. Te extracellular part of helices I and II move inwards, and the intracellular part of helix VI moves outwards, thereby shrinking the volume of the orthosteric ligand-binding site by 53%28. In addition, the conformational changes of a twin toggle switch of Phe200 and Trp356 were also observed. 1Center for Supercomputing and Big Data, DGIST, 333 Techno jungang-daero, Daegu, 42988, Korea. 2Department of Bioinformatics, Korea University, 2511 Sejong-ro, Sejong, 30019, Korea. 3Department of Brain and Cognitive Sciences, DGIST, 333 Techno jungang-daero, Daegu, 42988, Korea. 4Core Protein Resources Center, DGIST, 333 Techno jungang-daero, Daegu, 42988, Korea. Correspondence and requests for materials should be addressed to A.E.C. (email: [email protected]) or W.Y. (email: [email protected]) SCIENTIFIC REPORTS | (2018) 8:13787 | DOI:10.1038/s41598-018-31749-z 1 www.nature.com/scientificreports/ Tese fndings provide new insights into the mechanisms of structural changes depending on two classes of ligands and how they are bound in the orthosteric ligand-binding site. Although the crystal structures of CB1 have been determined, a large amount of work still needs to be done in order to understand the dynamic behaviors of the two conformations of CB1 as well as to be able to design chemically diverse ligands with distinct efcacy profles. Here, we demonstrate the dynamic behaviors of intact CB1 structures when the three classes of ligands were bound to the active and inactive conformations for the discrimination of ligand efcacy through molecular dynamics (MD) simulations. One study by West et al. experimentally demonstrated that the distinct confor- 29 mational states of beta-2-adrenergic receptor (β2AR) were induced by the diverse classes of ligands . When the ligands were bound to the β2AR, the intra- and extracellular regions were notably changed and showed distinct conformations depending on the ligands. Tus, to investigate the dynamic behaviors of CB1 structure, the active and inactive conformations of CB1 structures were used to identify the structural rearrangement induced by ligand binding. Tree classes of ligands, including THC as a partial agonist, THCV as an antagonist, and tarana- bant as an inverse agonist, were docked to the two structures. With these complex structures, MD simulations were carried out. In addition, the molecular mechanics Poisson-Boltzmann surface area (MM-PBSA) method was used to examine the binding energies of the three classes of ligands in the two conformations of CB1 and to determine the residual contributions of ligand binding. Our fndings will help to discriminate the distinct efcacy profles of the ligands and to provide new opportunities for the design of new CB1 drugs. Computational Methods Protein preparation. Te crystal structures for the inactive and active conformations of CB1 (PDB ID: 5TGZ26 and 5XRA28) were obtained from the Protein Data Bank (PDB)30,31. Both structures were modifed by mutating residues and inserting favodoxin into the ICL3 region to facilitate crystallization. In order to per- form molecular dynamics simulations, inactive and active conformations of wild-type intact CB1 structures were generated by reverting the mutant residues to wild-type and by reconstructing the ICL3 region using Modeller v9.1832,33, which was used in several studies for modelling ICL3 region in GPCRs34,35. A total of 20 structures for each conformation of CB1 were generated, and the one with the lowest discrete optimized protein energy (DOPE) score was selected. A loop refnement step was then performed to generate the 10 diferent loop structures, and the one adopted unstructured conformation with the lowest DOPE score was fnally selected. Te two fnal structures, including the active and inactive conformations of the wild-type intact CB1 struc- tures, were prepared using the protein preparation wizard36,37 module of the Schrödinger suite. Te protonation and tautomeric states of Asp, Glu, Arg, Lys, and His residues were adjusted to match a pH of 7.0. Te possible orientations of the Asn and Gln residues were generated. Finally, restrained minimization with the OPLS_2005 force feld38 was performed with the hydrogens only option to optimize the hydrogen atom positions. Ligand preparation. All three ligand structures for Δ9-tetrahydrocannabinol (THC), Δ9-tetrahydrocannabivarin (THCV), and taranabant were initially drawn using the 2D-Sketcher and prepared using the LigPrep39 module of Schrödinger suite with the OPLS_2005 force feld. LigPrep generated tautomers and stereoisomers within a pH range of 7.0 ± 2.0 using Epik40–42. Only the lowest energy conformer was retained for each ligand. Next, the ligands were opti- mized using the Jaguar43,44 module of the Schrödinger suite at the B3LYP/6–31 G* basis set. Te calculated electrostatic potential (ESP) charges were used as partial charges for the ligands. Molecular docking. Te three ligands were docked to the orthosteric binding site of the inactive confor- mation of the CB1 structure while two ligands, THC and THCV, were docked to the same site of the active conformation of the CB1 structure by using the Glide45–47 module of Schrödinger suite. Glide uses grids for fast scoring; the grid-generation module was used to generate grids for the two conformations of the CB1 structures. Te van der Waals (vdW) scaling and partial charge cutof was set to 0.8 and 0.15, respectively. Next, the SP mode of Glide was used to produce 5 poses per ligand, and the one pose with the lowest docking score was selected. In addition, the induced-ft docking (IFD)48,49 module of Schrödinger suite was used to dock the taranabant to the active conformation of the CB1 structure using default parameters, and the one pose with the lowest docking score was selected. System setup. A total of eight structures were used for the simulations: six CB1-ligand complex struc- tures from the docking simulations as well as the inactive and active apo CB1 structures. Te orientation of the CB1 structures with respect to the membrane were determined by using the Positioning of Proteins in Membrane (PPM) server of the Orientations of Proteins and Membranes (OPM) database50. Te oriented pro- teins were inserted in the 1-palmitoyl-2-oleoyl-sn-glycero-3-phosphocholine (POPC) lipid bilayer using the CHARMM-GUI Membrane Builder51–53. Te protein-membrane system was solvated with TIP3P54 water and 0.15 M NaCl. Te fnal system size was approximately 79 Å × 79 Å × 111 Å in the inactive conformation and 88 Å × 88 Å × 116 Å in the active conformation.
Details
-
File Typepdf
-
Upload Time-
-
Content LanguagesEnglish
-
Upload UserAnonymous/Not logged-in
-
File Pages11 Page
-
File Size-