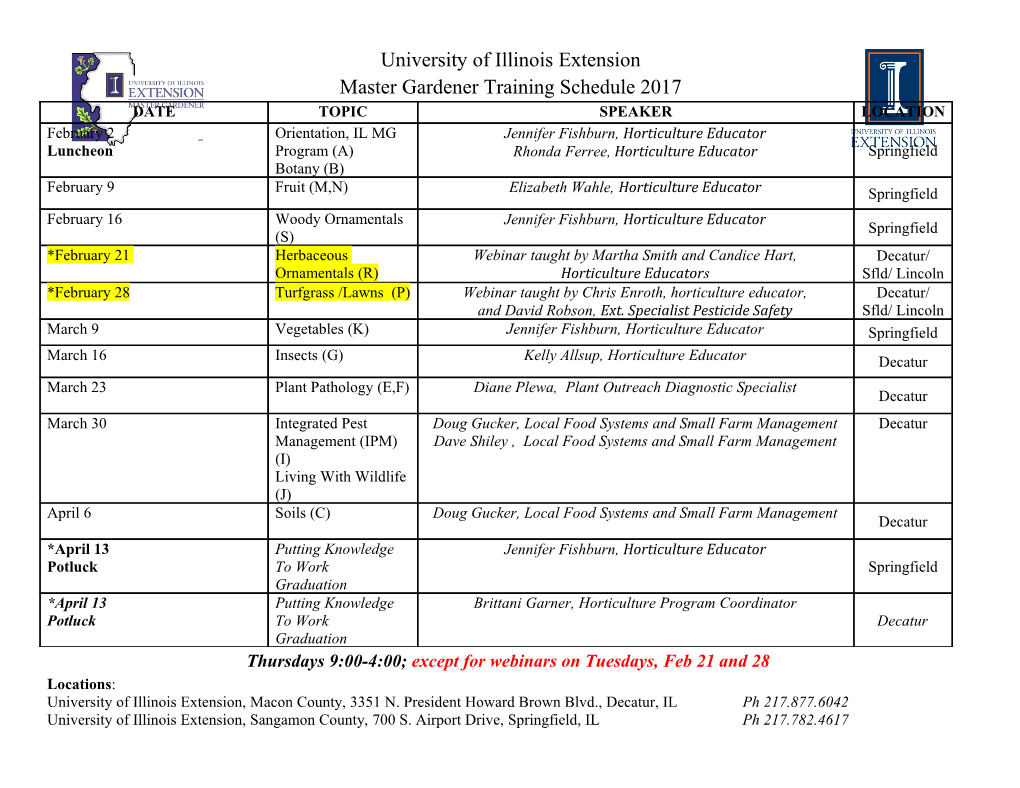
7 Physical Climate Processes and Feedbacks Co-ordinating Lead Author T.F. Stocker Lead Authors G.K.C. Clarke, H. Le Treut, R.S. Lindzen, V.P. Meleshko, R.K. Mugara, T.N. Palmer, R.T. Pierrehumbert, P.J. Sellers, K.E. Trenberth, J. Willebrand Contributing Authors R.B. Alley, O.E. Anisimov, C. Appenzeller, R.G. Barry, J.J. Bates, R. Bindschadler, G.B. Bonan, C.W. Böning, S. Bony, H. Bryden, M.A. Cane, J.A. Curry, T. Delworth, A.S. Denning, R.E. Dickinson, K. Echelmeyer, K. Emanuel, G. Flato, I. Fung, M. Geller, P.R. Gent, S.M. Griffies, I. Held, A. Henderson-Sellers, A.A.M. Holtslag, F. Hourdin, J.W. Hurrell, V.M. Kattsov, P.D. Killworth, Y. Kushnir, W.G. Large, M. Latif, P. Lemke, M.E. Mann, G. Meehl, U. Mikolajewicz, W. O’Hirok, C.L. Parkinson, A. Payne, A. Pitman, J. Polcher, I. Polyakov, V. Ramaswamy, P.J. Rasch, E.P. Salathe, C. Schär, R.W. Schmitt, T.G. Shepherd, B.J. Soden, R.W. Spencer, P. Taylor, A. Timmermann, K.Y. Vinnikov, M. Visbeck, S.E. Wijffels, M. Wild Review Editors S. Manabe, P. Mason Contents Executive Summary 419 7.3 Oceanic Processes and Feedbacks 435 7.3.1 Surface Mixed Layer 436 7.1 Introduction 421 7.3.2 Convection 436 7.1.1 Issues of Continuing Interest 421 7.3.3 Interior Ocean Mixing 437 7.1.2 New Results since the SAR 422 7.3.4 Mesoscale Eddies 437 7.1.3 Predictability of the Climate System 422 7.3.5 Flows over Sills and through Straits 438 7.3.6 Horizontal Circulation and Boundary 7.2 Atmospheric Processes and Feedbacks 423 Currents 439 7.2.1 Physics of the Water Vapour and Cloud 7.3.7 Thermohaline Circulation and Ocean Feedbacks 423 Reorganisations 439 7.2.1.1 Water vapour feedback 425 7.2.1.2 Representation of water vapour in 7.4 Land-Surface Processes and Feedbacks 440 models 425 7.4.1 Land-Surface Parametrization (LSP) 7.2.1.3 Summary on water vapour Development 440 feedbacks 426 7.4.2 Land-Surface Change 443 7.2.2 Cloud Processes and Feedbacks 427 7.4.3 Land Hydrology, Runoff and Surface- 7.2.2.1 General design of cloud schemes Atmosphere Exchange 444 within climate models 427 7.2.2.2 Convective processes 428 7.5 Cryosphere Processes and Feedbacks 444 7.2.2.3 Boundary-layer mixing and 7.5.1 Snow Cover and Permafrost 444 cloudiness 428 7.5.2 Sea Ice 445 7.2.2.4 Cloud-radiative feedback processes 429 7.5.3 Land Ice 448 7.2.2.5 Representation of cloud processes in models 431 7.6 Processes, Feedbacks and Phenomena in the 7.2.3 Precipitation 431 Coupled System 449 7.2.3.1 Precipitation processes 431 7.6.1 Surface Fluxes and Transport of Heat and 7.2.3.2 Precipitation modelling 431 Fresh Water 449 7.2.3.3 The temperature-moisture feedback 7.6.2 Ocean-atmosphere Interactions 451 and implications for precipitation 7.6.3 Monsoons and Teleconnections 451 and extremes 432 7.6.4 North Atlantic Oscillation and Decadal 7.2.4 Radiative Processes 432 Variability 452 7.2.4.1 Radiative processes in the 7.6.5 El Niño-Southern Oscillation (ENSO) 453 troposphere 432 7.6.5.1 ENSO processes 454 7.2.4.2 Radiative processes in the 7.6.5.2 ENSO and tropical storms 455 stratosphere 433 7.2.5 Stratospheric Dynamics 434 7.7 Rapid Changes in the Climate System 455 7.2.6 Atmospheric Circulation Regimes 435 7.2.7 Processes Involving Orography 435 References 457 Physical Climate Processes and Feedbacks 419 Executive Summary the distribution of water vapour. The physical basis of the cloud parametrizations included into the models has also been greatly Considerable advances have been made in the understanding of improved. However, this increased physical veracity has not processes and feedbacks in the climate system. This has led to a reduced the uncertainty attached to cloud feedbacks: even the better representation of processes and feedbacks in numerical sign of this feedback remains unknown. A key issue, which also climate models, which have become much more comprehensive. has large implications for changes in precipitation, is the Because of the presence of non-linear processes in the climate sensitivity of sub-grid scale dynamical processes, turbulent and system, deterministic projections of changes are potentially convective, to climate change. It depends on sub-grid features of subject to uncertainties arising from sensitivity to initial surface conditions such as orography. Equally important are conditions or to parameter settings. Such uncertainties can be microphysical processes, which have only recently been partially quantified from ensembles of climate change integra- introduced explicitly in the models, and carry major uncertain- tions, made using different models starting from different initial ties. The possibility that models underestimate solar absorption in conditions. They necessarily give rise to probabilistic estimates clouds remains controversial, as does the effect of such an of climate change. This results in more quantitative estimates of underestimate on climate sensitivity. The importance of the uncertainties and more reliable projections of anthropogenic structure of the stratosphere and both radiative and dynamical climate change. While improved parametrizations have built processes have been recognised, and limitations in representing confidence in some areas, recognition of the complexity in other stratospheric processes add some uncertainty to model results. areas has not indicated an overall reduction or shift in the current Considerable improvements have taken place in modelling range of uncertainty of model response to changes in atmospheric ocean processes. In conjunction with an increase in resolution, composition. these improvements have, in some models, allowed a more Atmospheric feedbacks largely control climate sensitivity. realistic simulation of the transports and air-sea fluxes of heat and Important progress has been made in the understanding of those fresh water, thereby reducing the need for flux adjustments in processes, partly by utilising new data against which models can coupled models. These improvements have also contributed to be compared. Since the Second Assessment Report (IPCC, 1996) better simulations of natural large-scale circulation patterns such (hereafter SAR), there has been a better appreciation of the as El Niño-Southern Oscillation (ENSO) and the oceanic complexity of the mechanisms controlling water vapour distribu- response to atmospheric variability associated with the North tion. Within the boundary layer, water vapour increases with Atlantic Oscillation (NAO). However, significant deficiencies in increasing temperatures. In the free troposphere above the ocean models remain. Boundary currents in climate simulations boundary layer, where the greenhouse effect of water vapour is are much weaker and wider than in nature, though the most important, the situation is less amenable to straightforward consequences of this fact for the global climate sensitivity are not thermodynamic arguments. In models, increases in water vapour clear. Improved parametrizations of important sub-grid scale in this region are the most important reason for large responses to processes, such as mesoscale eddies, have increased the realism increased greenhouse gases. of simulations but important details are still under debate. Major Water vapour feedback, as derived from current models, uncertainties still exist with the representation of small-scale approximately doubles the warming from what it would be for processes, such as overflows and flow through narrow channels fixed water vapour. Since the SAR, major improvements have (e.g., between Greenland and Iceland), western boundary occurred in the treatment of water vapour in models, although currents (i.e., large-scale narrow currents along coastlines), detrainment of moisture from clouds remains quite uncertain and convection, and mixing. discrepancies exist between model water vapour distributions and In the Atlantic, the thermohaline circulation (THC) is those observed. It is likely that some of the apparent discrepancy responsible for the major part of the ocean meridional heat is due to observational error and shortcomings in intercompar- transport associated with warm and saline surface waters flowing ison methodology. Models are capable of simulating the moist northward and cold and fresh waters from the North Atlantic and very dry regions observed in the tropics and sub-tropics and returning at depth. The interplay between the large-scale how they evolve with the seasons and from year to year, atmospheric forcing, with warming and evaporation in low indicating that the models have successfully incorporated the latitudes, and cooling and net precipitation at high latitudes, basic processes governing water vapour distribution. While forms the basis of a potential instability of the present Atlantic reassuring, this does not provide a definitive check of the THC. Changes in ENSO may also influence the Atlantic THC by feedbacks, though the balance of evidence favours a positive altering the fresh water balance of the tropical Atlantic, therefore clear-sky water vapour feedback of a magnitude comparable to providing a coupling between low and high latitudes. Uncertainty that found in simulations. resides with the relative importance of feedbacks associated with Probably the greatest uncertainty in future projections of processes influencing changes in high latitude sea surface temper- climate arises from clouds and their interactions with radiation. atures and salinities, such as atmosphere-ocean heat and fresh Cloud feedbacks depend upon changes in cloud height, amount, water fluxes, formation and transport of sea ice, continental runoff and radiative properties, including short-wave absorption. The and the large-scale transports in ocean and atmosphere. The radiative properties depend upon cloud thickness, particle size, Atlantic THC is likely to change over the coming century but its shape, and distribution and on aerosol effects. The evolution of evolution continues to be an unresolved issue.
Details
-
File Typepdf
-
Upload Time-
-
Content LanguagesEnglish
-
Upload UserAnonymous/Not logged-in
-
File Pages54 Page
-
File Size-