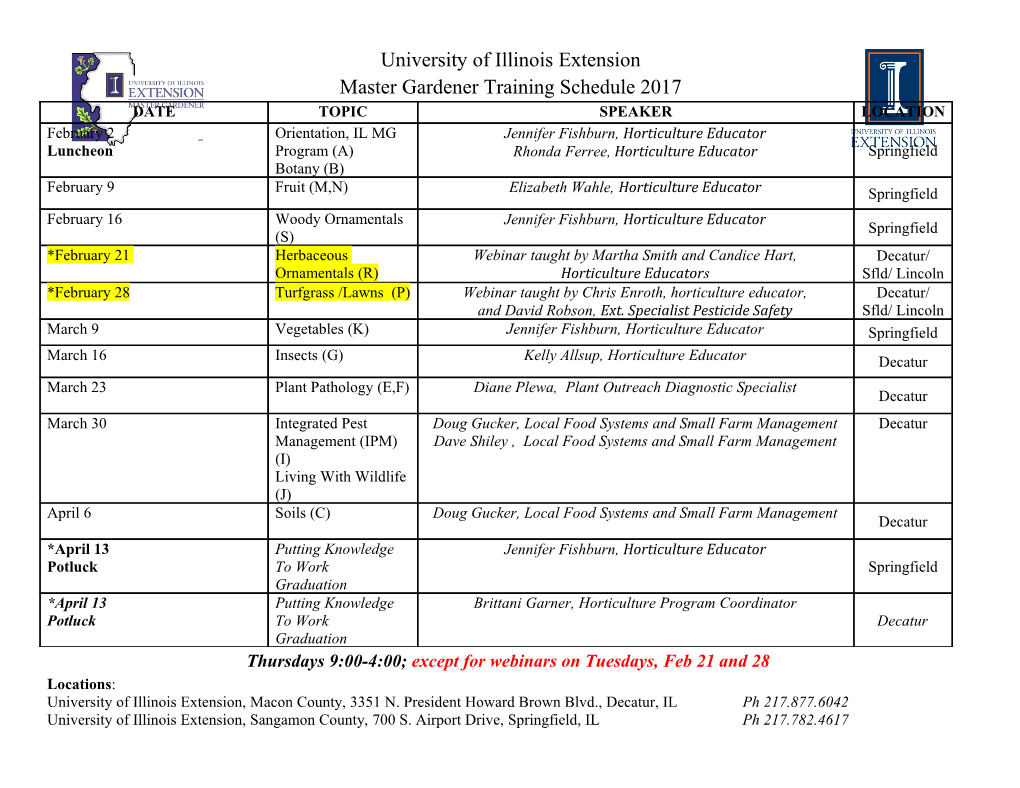
Pollen wall development in mango (Mangifera indica L., Anacardiaceae) Jorge Lora and José I. Hormaza Department of Subtropical Fruit Crops. Instituto de Hortofruticultura Subtropical y Mediterránea La Mayora (IHSM la Mayora-UMA-CSIC). Avenida Dr. Wienberg, s/n. 29750 Algarrobo-Costa, Málaga (Spain) Tel: (+34) 952 54 89 90 Fax:(+34) 952 55 26 77 ✉ Jorge Lora [email protected] ORCID ID: 0000-0001-9713-0431 1 Abstract The mango (Mangifera indica) is a woody perennial crop currently cultivated worldwide in regions with tropical and subtropical climates. Despite its importance, an essential process such as pollen development, and, specifically, cell wall composition that influences cross-talk between somatic cells and the male germline, is still poorly understood in this species and in the Anacardiaceae as a whole. A detailed understanding of this process is particularly important to know the effect of low temperatures during flowering on pollen development that can be a limiting factor for fertilization and fruit set. To fill this gap, we performed a thorough study on the cell wall composition during pollen development in mango. The results obtained reveal a clear differentiation of the cell wall composition of the male germline by pectins, AGPs and extensins from the early developmental stages during microsporogenesis and microgametogenesis reflecting a restricted communication between the male germline and the surrounding somatic cells that is very sensitive to low temperatures. The combination of the results obtained provides an integrated study on cell wall composition of the male germline in mango that reveals the crucial role of the sporophyte and the gametophyte and the vulnerability of the process to low temperatures. Keywords Anacardiaceae, cell wall, low temperature, Mangifera indica, mango, pollen development. 2 Introduction The mango (Mangifera indica L.) is one of the five most important fruit crops worldwide (together with bananas, oranges, grapes and apples) reaching a production of nearly 50 million tons in 2016 (FAO 2017). Probably mango was domesticated at least 6000 years ago (Mukherjee and Litz 2009) independently in different regions of Asia (Bompard 2009), resulting in two different ecogeographic races: monoembryonic mangoes from subtropical India and polyembryonic mangoes from southeastern tropical Asia. Currently, India is the largest producer of mango, contributing 34% of the world´s total production and, together with other Asian countries such as China, Thailand and Indonesia, account for more than 70% of global mango production (FAO 2017). Mango cultivation has also been spread to other tropical and subtropical regions and can be found in over 90 countries (Evans and Mendoza 2009). Systematic collection and crosses of mango genetic resources in south Florida resulted in the release of several new cultivars that currently dominate international trade and, in fact, Florida is now considered as a secondary center of mango diversity (Viruel et al. 2005; Mukherjee and Litz 2009). The genus Mangifera belongs to the family Anacardiaceae in the Sapindales with other species of agronomic interest such as pistachio (Pistacia vera L.) or cashew (Anacardium occidentale L.) among their 73 genera and 850 species (Bompard 2009). The genus Mangifera contains 69 species and it is subdivided in two subgenera, Limus and Mangifera (Kostermans et al. 1993). Due to its agronomic interest the mango has been subjected to extensive studies mainly focused on optimizing tree management and improving yield. In the reproductive phase, several studies have been performed on the effect of the environment on pollen germination (de Wet and Robbertse 1986; de Wet et al. 1989; Dag et al. 2000) and flowering (Ramírez and Davenport 2010), important factors for production since mango trees show a low fruit set generally lower than 0.1% under open pollination (Singh 1960). The mango is an andromonoecious tree with a variable proportion of hermaphrodite and male flowers borne in terminal inflorescences which are composed thirsoids (Coetzer et al. 1995). The number of flowers and the ratio of hermaphrodite to male flowers varies depending on the cultivar, climatic conditions, location in the tree, and the production in the previous season (Mukherjee and Litz 2009). Low temperatures affect fertilization and fruit set and, as a consequence, a usual practice in countries such as Spain and Israel, in which a first bloom occurs with too low night temperatures, is to delay the flowering season by removing the first inflorescences produced (Dag et al. 2000; Torres et al. 2009). However, despite the commercial importance of mango and other crops of the Anacardiaceae, an essential process in sexual reproduction such as pollen development has been slightly studied in this family (Maheshwari 1934; Srinivasachar 1940; Kelkar 1958; Copeland 1959; Issarakraisila and Considine 1994; Oliveira and Mariath 2001; Huang et al. 2010). Those studies have been mainly focused on pollen morphology from a taxonomic point of view (Ibe and Leis 1979; Belhadj et al. 2007; Pell et al. 2010) and on pollen germination from a agronomical point of view (de Wet and Robbertse 1986; de Wet et al. 1989; Wunnachit et al. 1992; Issarakraisila and Considine 1994; Dag et al. 2000; Huang et al. 2010; Ramírez and Davenport 2016). Issarakraisila and Considine (1994) were the first to report the best temperature range for mango pollen development, showing that the phase from meiosis to early microspore development was very sensitive to low temperatures. Similar conclusions were also obtained in a later study (Huang et al. 2010). Pollen development is a complex and highly conserved process in angiosperms (McCormick 2004; Blackmore et al. 2007) that has been widely studied in model plants such as Arabidopsis thaliana or rice (Oryza sativa) in which the molecular and genetic pathways involved 3 in pollen development have been partially revealed (Wilson and Zhang 2009; Gómez et al. 2015; Shi et al. 2015). During pollen development, cell wall composition influences cell-cell communication and, therefore, is likely to influence cross-talk between the somatic and the reproductive cells. It is specifically important during microsporogenesis (the transition from somatic to the germ line cell, the arquesporial cell) and later, when the arquesporial cell increases in size becoming the microspore mother cell, that undergoes two meiotic divisions to form four haploid microspores (microspore tetrad). During this period an intense cell-cell communication takes place between the cells of the male germ line and the surrounding somatic cells. Moreover, the pollen cell wall is the most complex structure among plant cell walls with an outer and inner walls, the exine and the intine, respectively (Blackmore et al. 2007). The exine is more complex and consists of an outer layer, sexine, and an inner nexine (Heslop-Harrison 1968). The exine generally includes material from the tapetum and is composed mostly by a combination of polymers that forms the sporopollenin (Blackmore et al. 2007; Quilichini et al. 2015). Recent studies have reported that the inner layer, nexine, is composed of arabinogalactan proteins (AGPs) in Arabidopsis thaliana (Lou et al. 2014, Jia et al. 2015). Indeed, previous functional studies identified the genes encoding AGPs and their essential role in pollen development in Arabidopsis (Levitin et al. 2008; Coimbra et al. 2009) that were also observed around cells in the reproductive lineage and in the intine (Coimbra et al. 2007). AGPs have also been observed in the intine of an eudicot, Quercus suber (Costa et al. 2015), and of the early divergent Trithuria submersa (Costa et al. 2013). Other component of the pollen cell wall are cellulose and non-cellulosic polysaccharides such as pectins that are the main components of the intine (Heslop-Harrison 1968; Blackmore et al. 2007), and also of the plant cell wall as a whole (Palin and Geitmann 2012). Pectins together with callose, a polysaccharide that is also found around the microspore mother cells and may act as a molecular filter, have also been revealed to play an important role on the apertures and on the pollen dispersal unit in the genus Annona (Lora et al. 2009, 2014). While the pollen development process has been described in several plant species, detailed studies on changes in cell wall components during pollen development are scarce (Coimbra et al. 2007; Lora et al. 2009, 2014, Costa et al. 2013, 2015) and, to the best of our knowledge, absent in the Anacardiaceae. Thus, in this work we perform an integrated study on pollen development in M. indica, giving special attention to the cell wall composition, and specifically to pectins, AGPs, extensins and cellulose. Moreover, we evaluate the effect of low temperatures on the cell wall composition during pollen development of the first bloom under Mediterranean conditions. Material and methods Plant material Adult trees of two M. indica cultivars, M. indica `Kensington´ and M. indica `Kent´ located in a field germplasm collection at the IHSM La Mayora-CSIC, Málaga, Spain, were used for this work, which was performed during the first and second flowering periods in March and May, respectively, of 2017. Light microscopy 4 To follow pollen development, we collected anthers from flower buds at a range of developmental stages, from differentiation up to anther dehiscence. The anthers were fixed in 2.5% v/v glutaraldehyde in 0.03 M phosphate buffer (Sabatini et al. 1963), dehydrated
Details
-
File Typepdf
-
Upload Time-
-
Content LanguagesEnglish
-
Upload UserAnonymous/Not logged-in
-
File Pages22 Page
-
File Size-