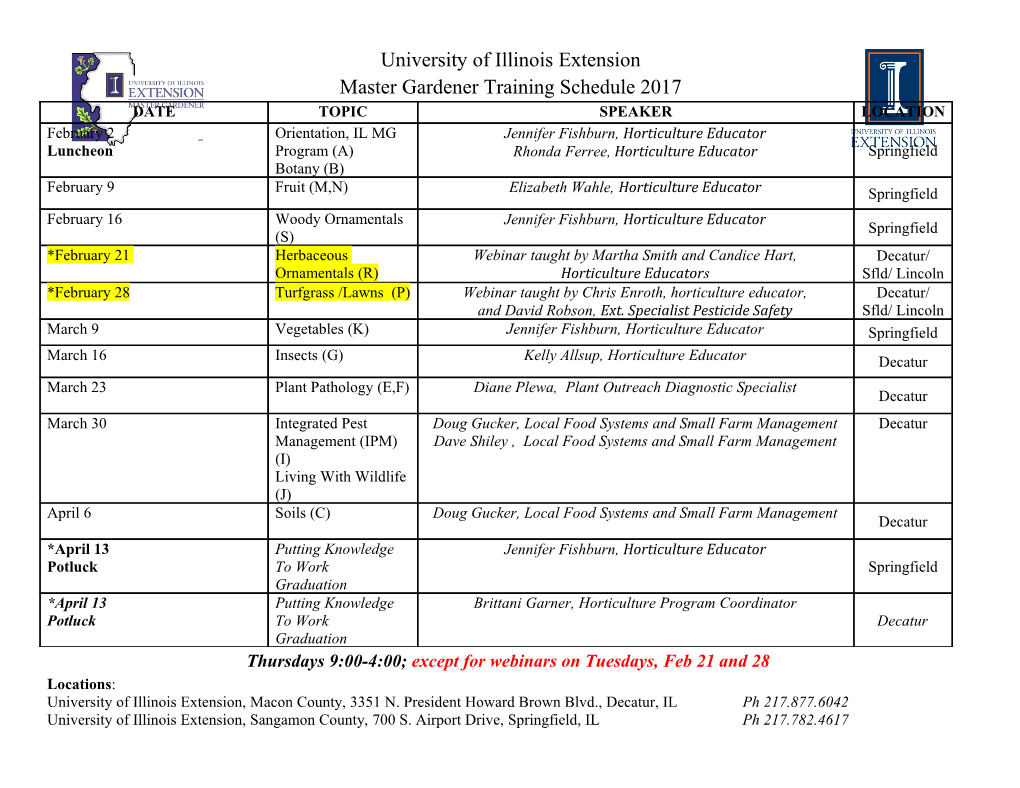
No. 5] Proc. Jpn. Acad., Ser. B 97 (2021) 211 Review Experimental verification of the standard model of particle physics † By Tomio KOBAYASHI*1, (Edited by Takaaki KAJITA, M.J.A.) Abstract: The history concerning an experimental verification of the standard model of particle physics is reviewed with special emphasis on results from experiments using the highest- energy particle colliders, namely, PETRA, LEP and LHC. This article covers physics subjects from discovering the gluon and precise measurements at LEP, to discovering the Higgs boson. It also covers some searches for physics beyond the standard model, particularly supersymmetry, as well as recent developments of some particle detectors that were used in those experiments. Keywords: standard model, gluon, Higgs boson, supersymmetry 1. Introduction Table 1. Fermions in the standard model as of 1974 1-st 2-nd 3-rd charge All theoretical ingredients for the standard particle model of particle physics were available by 1974, generation generation generation (e) and were ready to be scrutinized by experiments. up-type uct2/3 During 40 years thereafter, a number of discoveries quark and fine measurements were made, and the standard down-type dsb!1/3 model became established. quark The standard model of particle physics is based neutrino 8e 87 8= 0 on theories that describe the elementary particles and charged e 7=!1 their interactions, namely the electro-magnetic, weak lepton and strong forces. Particle charges are in the unit of the elementary charge (e). The present particle contents of the standard Particles in the higher generation are heavier than the ones in model are given in Table 1 (fermions) and in Table 2 the lower generation, except that neutrinos are assumed to be (bosons). The particles in the hatched areas were not massless. The particles in hatched area were not found until 1974. found before 1974. Fermions (spin 1/2) were sup- posed to be the basic constituents of all material in Table 2. Bosons in the standard model as of 1974 the universe. Vector bosons (spin 1) are carriers of 2 the basic forces of the standard model, and a scaler particle spin charge (e) mass (GeV/c ) boson (spin 0) is responsible for the mass of all photon . 10 0 particles in the standard model. gluon g 10 0 The fundamental theory of the standard model charged weak boson W 1 ’1 80.385 is the gauge theory. The electro-magnetic interaction neutral weak boson Z 1 0 91.188 is described by the U(1) gauge theory: quantum Higgs boson H 0 0 125.1 electrodynamics (QED). The electro-magnetic force The particles in hatched area were not found until 1974. and the weak force are combined into the electro- weak (EW) force in the SU(2) # U(1) gauge theory, giving a massless photon (.) and massive weak bosons (W ’, Z 0). The Higgs mechanism was *1 International Center for Elementary Particle Physics introduced in the EW theory, so as to give masses (ICEPP), The University of Tokyo, Hongo 7-3-1, Bunkyo-ku, to the standard-model particles, and as a conse- Tokyo 113-0033, Japan. † quence a spinless neutral Higgs boson (H) should Correspondence should be addressed: T. Kobayashi, ro- 358, Asahi, Chiba 289-2516, Japan (e-mail: [email protected] exist. The strong force between quarks, and hence tokyo.ac.jp). between hadrons, is described by SU(3) gauge theory doi: 10.2183/pjab.97.013 ©2021 The Japan Academy 212 T. KOBAYASHI [Vol. 97, with 3 color charges. This theory, called quantum DESY then decided to buildpffiffiffi the world highest chromodynamics (QCD), predicts the existence of energy eDe! collider, PETRA ( s ¼ 46:8 GeV max.), gluons as the carrier of the strong force. But none of of which one of the main objectives was to find the the bosons, except for the photon, were found before top quark (t), a counterpart of the b quark in the 3-rd 1974. generation quark doublet. PETRA completed its The fermionic part of the standard model construction and started operation in 1978. The consists of quarks, which have all 3 interactions ICEPP team formed the international group, the (i.e., electro-magnetic, weak and strong forces), and JADE collaboration, together with German and UK leptons, which have 2 interactions other than the teams to participate in experiments at PETRA. strong interaction. Quarks, as well as leptons, appear The author joined the ICEPP team as a research as a pair (like u and d, or 8e and e) to form an SU(2) staff member in 1977, and participated in the JADE doublet of the weak interaction. Fermions in the 1-st experiment. The activities of ICEPP continued from generation are supposed to form almost all of the experiments at DESY to two experiments at the materials in the universe, since stable atoms or nuclei European Organization for Nuclear Research are made of them. (CERN): OPAL experiment using LEP eDe! collider Until 1974, all of the hadrons, including the from 1980, and ATLAS experiment using the LHC strange particles, were explained by the quark model proton-proton collider from 1992. The author was with 3 quarks (u, d and s). While the lepton doublet involved in all 3 experiments (JADE, OPAL and of the 2-nd generation (87, 7) was already found, the ATLAS) until 2015. counterpart of the s-quark was missing. The GIM This article describes the important findings mechanism1) was proposed to cure a strangeness- made by the experiments at PETRA, LEP and LHC, changing neutral-current problem, which predicted as well as those from other experiments, in the light the existence of a 4-th quark (charm, c). of an experimental verification of the standard model In November 1974, the J/A particle was of particle physics. discovered at Brookhaven National Laboratory The standard model was known to have its own (BNL) and at Stanford Linear Accelerator Center limitation, namely: i) it does not have the gravity (SLAC). Soon afterwards the J/A was confirmed to interaction, ii) it has a charge quantization problem, be a ground-state charmonium, which is a meson iii) too many parameters, iv) a hierarchy problem, composed of c and c (anti-particle of c quark). The v) a generation problem, vi) no solutions to dark other charmonium states as well as the open charm matter, dark energy, and baryon asymmetry in the states (both charmed mesons and charmed baryons) universe, and so on. These necessitate a new theory were discovered one after another, so that the which includes the standard model. apparent flaw of the standard model framework had There are a number of theories which can solve been removed. Hence, the discovery of J/A was called some of these problems. Some of the candidates are: the “November Revolution”, and it was the beginning theories with supersymmetry, quark/lepton substruc- of an experimental verification of the standard model tures, technicolor models, quantum gravity theories, of particle physics. etc. During the course of experimentally verifying In 1975 the = lepton was discovered at SLAC, the standard model of particle physics, especially the and in 1977 the Ç particle (the ground-state supersymmetry theories and the technicolor theories, bottomonium bb) was discovered at Fermi National these came up as the main candidate theories just Accelerator Laboratory (Fermilab), which strongly beyond the standard model. In this article some of indicated the existence of 3-rd generation fermions. the searches for new physics beyond the standard The International Center for Elementary Par- model are also presented, with emphasis on these ticle Physics (ICEPP) was established in 1974 at the two types of theories. The details of these theories University of Tokyo in order to participate in the are briefly described in the relevant sections of this DASP experiment using the DORIS accelerator at article. Deutsches Elektronen Synchrotron (DESY). DORIS D ! 2. PETRA period (1978–) is a positron-electron (e e ) collider of whichpffiffiffi the maximum center-of-mass (c.m.) energy ( s) was The PETRA (Positronen-Electronen Tandem 11.2 GeV. The ICEPP team made an essential Ring Anlage) eDe! collider was constructed at contribution to the discovery of the Pc particle, one DESY, and started operation in 1978. By thep endffiffiffi of the charmonium states. of 1979, it provided eDe! collisions between s ¼ No. 5] Experimental verification of the standard model of particle physics 213 Fig. 1. Sectional view of the JADE detector. 12 GeV and 31.6 GeV, which gave rise to many New flavor quark pair production in eDe! important results. PETRA continued working until collisions should enhance the multihadronic event 1986, and its attained highest collision energy was rate, so that the search was made through measure- 46.8 GeV. ments of the R value: There were 4 experiments at PETRA: JADE, ðeþeÀ ! hadronsÞ Mark J, TASSO and PLUTO (which was replaced by R ¼ : ½1 ðeþeÀ ! þÀÞ CELLO from 1980). A sectional view of the JADE P 3 2 detector is shown in Fig. 1. The ICEPP team was It is equal to i qi in the simple quark model, involved in the JADE experiment, and contributed where qi is the charge (in the unit of e) of the quark i, to the construction and operation of the electro- and the factor 3 comes from the number of quark magnetic shower calorimeter, which consisted of 2520 colors. The results were consistent with the produc- lead glass counters in the barrel part, and 192 lead tion of the known 5 quarks (u, d, s, c, b), and there glass counters on the two end caps of the detector. was no sign of any excess concerning the R values.
Details
-
File Typepdf
-
Upload Time-
-
Content LanguagesEnglish
-
Upload UserAnonymous/Not logged-in
-
File Pages25 Page
-
File Size-