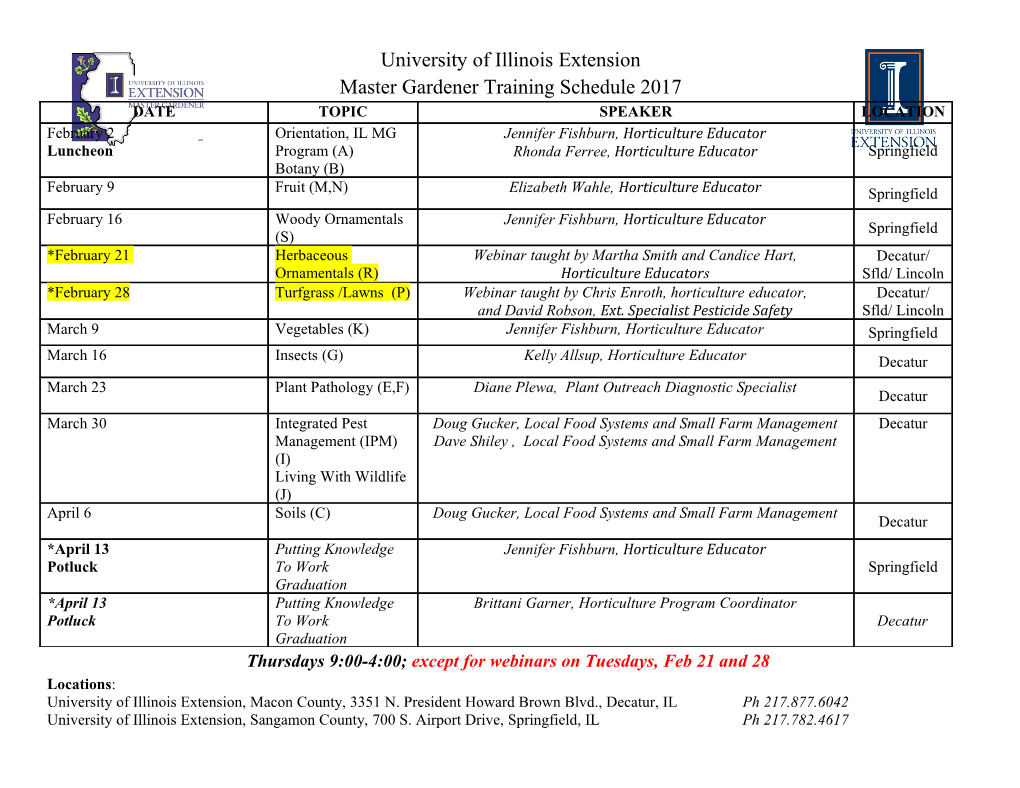
552.12:553.08:631.416.4/. MEDEDELINGEN LANDBOUWHOGESCHOOL WAGENINGEN • NEDERLAND • 80-16(1980) RELATIVE STABILITIES OF SOIL MINERALS (with a summary in English) E. L. MEIJER and L. VAN DER PLAS Department of Soil Science and Geology, Agricultural University, Wageningen, The Netherlands (Received 5-IX-1980) H. VEENMAN & ZONEN B.V. - WAGENINGEN - 1980 RELATIVE STABILITIES OF SOIL MINERALS INTRODUCTION The stability of soil minerals is frequently considered in the geochemical literature (WILDMAN et al., 1971; KITTRICK, 1971a, 1971b; RAIan d LINDSAY, 1975; CURTIS, 1976; WEAVER et al., 1976; REESMAN, 1978). In most cases the possible composition of the aqueous solution coexisting with acertai n mineral is discussed in detail, thecompositio n being given in terms of activities ofth e relevant species in solution. The Gibbs free-energy change ofsuc h a reversible dissolution equilibrium is zero ifa n infinitely small amount ofth e mineral dissolves ((AG)R = 0). There­ fore, the mineral isonl y stable if for any other possible mineral made of com­ ponents present inth e system (AG)R ^ 0. It is quite understandable that the second part of this statement is rather difficult to investigate. The present paper will consider the importance ofthi s aspect. The Gibbs free-energy of formation of a mineral from itscomponent s ina well - defined system, e.g.,a n actual aqueous solution at 25° Can d 1bar , isindicate d by AG°el inthi s text. AG°el canb euse d to quantitatively describe the relative stability of a mineral. In doing so, three aspects have tob econsidere d : 1. The proper choice of molecular formula units enablinga compariso n of AG°el- values of minerals. 2. A proper reference system must be defined. 3. The chemical potentials ofth e relevant components have tob e defined. For example, BROWN and SKINNER (1974) calculated stabilities per cation accounting forth e first aspect. HUANG and KELLER (1972) suggested that the ideal muscovite 1 KAl2AlSi3O10(OH)2, AG° = -1313.8 kcal/mol isles s stable than a non-ideal muscovite K 84Ca08Mg 06Na01Fe"23Al2 32Si314A186O10(OH)2, AG° = -1399.9 kcal/mol. The AGf-values, however, have been calculated from equilibrium with the same solution. This means per definition that AG°el = 0fo r both minerals, i.e., both minerals areequall y stable inrelatio n toth ereferenc e system (cf. aspect 2). The differences in thevalue s of the Gibbs free-energy of formation from the elements does not imply that both minerals have different stabilities inth e soil system. WILDMAN etal . (1971) accounted explicitly forth e third aspect in concluding 'leal = 4.184J Meded. Landbouwhogeschool Wageningen 80-16 (1980) that Fe-rich montmorillonite is more stable than saponite in soils on serpentinites under certain conditions. REESMAN (1978) accounted for thesecon d andthir d aspect byspecifyin g five illite-equilibrated aqueous solutions, hedi dno tobserv e the first aspect. METHOD The following notation, illustrated with wollastonite, is preferred because it clearly shows therelatio n between theminera l andth ereferenc e system 2+ + CaSi03 -+ H4Si04 + Ca - 2H - H20 (1) Furthermore, there isn oreaso n tolimi t thechoic e ofth e reference system to (dilute) aqueous solutions. The actual soil system may well be rather dry or periodically wet; Therefore, the use of chemical potentials of the oxide components is used instead of activities in solution (cf. BROWN and SKINNER, 1974). This leads to CaSi03 -» CaO + Si02 (2) The relation at2 5°C and 1ba rbetwee n the oxide-potentials andth eactivitie s of the solved species follows from the hypothetical dissolution equilibria (cf. HELGESON, 1968, eq. (7)) + 2 + CaO + 2H «>Ca + H20 (3) Si02 + 2H 20 o H4Si04 (4) as: 2+ + AGf(CaO) = {AG?(Ca )aq - 2AG?(H )aq + AG?(H20)L} + (5.708 kJ/mol)• {log aCa2+ + 2p H + loga H2o} (5) AGf(Si02) = {AG?(H4Si04)aq - 2AG?(H20)L} + (5.708 kJ/mol) • {log aH4Si04 - 2lo ga H2o} (6) It follows from (5)an d(6 )tha t the chemical potentials ofCa O andSi0 2 area linear function of (log aCa2+ '+ 2pH ) and(lo ga H4Si04) only ifa H20 « 1. A quantitative measure ofminera l stability in a soil requires: 1.A proper choice ofth e formula unit 2. A definition of 'the chemical potentials of the oxides ina soil environment'. Aprope r formula unit iseasil ychosen .Th econventio n according towhic h the chemicalcompositio n ofmineral si sexpresse d interm so fon ecatio n per formula unit meets all the requirements, cf. NIGGLI (1936), VAN DER PLAS and VAN SCHUYLENBORGH (1970), BROWN and SKINNER (1974). H20 and C02 are not included in the total number of cations because they are exchangeable with 2 Meded. Landbouwhogeschool Wageningen 80-16 (1980) atmospheric H20 and C02. The chemical potentials of these components are therefore externally determined and not by the soil system. As to the second aspect, wemus t consider the question whether a Gibbs free- energyleve lca n befoun d for asoi lsyste m asa functio n ofchemica l composition. Moreprecisily ,ca nchemica lpotential s beattribute d toever yoxid ei nsuc ha way that for typical soil minerals AG°el x 0? Mathematically this means that the Gibbs free-energy of formation of a typical soil mineral can be calculated by linear summation of the chemical potentials of theconstituen t oxides.Suc h a linear combination can be envisaged as a 'Gibbs-plane' (BROWN and SKINNER, 1974). TARDY and GARRELS (1974) developed a comparable approach for the esti­ mation of Gibbs free-energies of formation of certain clay minerals. This ap­ proach has been extended to other types of silicates (TARDY and GARRELS,1977 ) and has been refined by NRIAGU (1975) and MATTIGOD and SPOSITO (1978). Surveying the sets of minerals for which estimation methods have been de­ veloped by the authorsjus t mentioned, it isclea r that other important groups of minerals have also to be considered in the soil system. A description of the minerals present in the Goethite-facies (VAN DER PLAS and VAN SCHUYLEN- BORGH, 1970) lists the following groups: oxides,hydroxides , phosphates, carbonates, hydrocarbonates, halides, sulfides, sulphates, the silicates belonging to the groups of smectites, hydrous micas, kandites, allophane, and the silica minerals quartz, low tridymite, low cristobalite. If we take a set of minerals consisting of members of these groups it follows after some calculation that the minerals of the set show a spread of only a few kJ/mol per ion2 from an average Gibbs free-energy level. For the selection of the minerals of the set (see table 1) the following obser­ vations have been used: 1. Asurve y ofth eGibb s free-energies offormatio n ofth erock - and soil-forming minerals shows a large amount of normalized values near to a specific low Gibbs free-energy level. 2. Only a small amount of minerals have normalized values significantly lower than this level, for example dolomite. 3. Anumbe r of minerals not belonging to the soilenvironmen t have normalized values of a significantly higher level. 4. For thedeterminatio n of areferenc e systemmineral so fgrou p 1 wereuse d for whichth edat a areinserte di nth erecen tdat a collection of ROBIEe tal .( 1978 )a s much as possible. 5. Theselecte dmineral swer euse d ina least-square s fitting procedure to develop mathematically a reference Gibbs-plane. Table 2 contains the fitted oxide-potentials. 2Cation san d CI" TABLE 1. Gibbs free-energies of formation of selected minerals and their deviations perio n from least-squares fitted values, at 298.15 K and 1 bar. 2 Mineral N'ions Formula AG? Ref AGüin kJ/mol kJ/mol Alunite 6 KA13(0H)6(S04)2 - 4656.58 1 + 1.8 Analbite 5 NaAlSi308 - 3706.507 2 + 1.0 Analcime 4 NaAlSi206-H20 - 3091.730 2 -1.1 Anhydrite 2 CaS04 - 1321.696 2 + 2.0 Arcanite 3 K2S04 - 1319.7 3 -0.8 Bischofite 3 MgCl2 •6H 20 - 2114.8 3 + 3.6 Bloedite 5 Na2Mg(S04)2 •4H 20 - 3430.1 3 -0.8 Boehmite 1 AIO(OH) - 918.400 2 -0.8 Calcit e 1 CaC03 - 1128.842 2 -0.4 Chrysotile 5 Mg3Si2Os(OH)4 - 4034.024 2 + 1.7 Epsomite 2 MgS04-7H20 - 2871.240 2 -1.2 Fayalite 3 Fe2Si04 - 1379.375 2 + 0.8 Gaylussite 3 Na2C03-CaC03-5H20 - 3372.7 3 + 0.6 Gibbsite 1 Al(OH)3 - 1154.889 2 -1.5 Glauberite 5 Na2Ca(S04)2 - 2596.590 4 -1.3 Goethite 1 FeO(OH) - 488.550 2 + 1.2 Gypsum 2 CaS04-2H20 - 1797.197 2 0.0 Halite 2 NaCl - 384.212 2 -2.9 Hematite 2 Fe203 - 742.683 2 + 0.6 Hercynite 3 FeAl204 - 1850.795 2 + 3.2 Huntite 4 CaMg3(C03)4 - 4203.425 2 -2.8 Hydromagnesite 5 5MgO-4C02-5H20 - 5864.166 2 + 3.3 Hydromagnesite 4 Mg4(C03)3(OH)2-3H20 - 4633.7 3 -2.5 Hydroxyapatite 8 Ca5(P04)3(OH) - 6338.434 2 -1.0 Kaliophillite 3 KAlSi04 - 2005.975 2 + 3.9 Kaolinite 4 Al2Si205(OH)4 - 3799.364 2 -2.0 Leonhardite 14 Ca2Al4Si8024-7H20 -13197.115 2 -1.3 Leucite 4 KAlSi206 - 2875.890 2 + 0.5 Low-albite 5 NaAlSi3Os - 3711.722 2 0.0 Magnetite 3 Fe304 - 1012.566 2 -2.7 Melanterite 2 FeS04 •7H 20 - 2509.641 2 + 1.5 Microcline 5 KAlSi308 - 3742.330 2 -0.9 Nahcolite 1 NaHC03 - 851.86 5 + 1.9 Natron 2 Na2CO3-10H2O - 3428.98 5 -2.0 Pirssonite 3 Na2C03-CaC03-2H20 - 2661.8 3 + 1.8 Pyrophyllite 6 Al2Si4Ol0(OH)2 - 5269.384 •2 + 1.1 Rutile 1 Ti02 - 889.446 2 — Sanidine 5 KAlSi308 - 3739.776 2 -0.4 Siderite 1 FeC03 - 666.698 2 -1.5 Sylvite 2 KCl - 408.554 2 -1.9 Talc 7 Mg3Si4O10(OH)2 - 5536.048 2 -1.3 Thenardite 3 Na2S04 - 1269.985 2 -1.8 Tremolit e 15 Ca2Mg5(Si8022)(OH)2 -11627.910 2 + 1.4 Trôna 3 Na2C03 •NaHC0 3 •2H 20 - 2386.55 5 + 2.0 Whitlockite 5 Ca3(P04)2 - 3895.654 2 + 0.9 1 See page3 .
Details
-
File Typepdf
-
Upload Time-
-
Content LanguagesEnglish
-
Upload UserAnonymous/Not logged-in
-
File Pages19 Page
-
File Size-