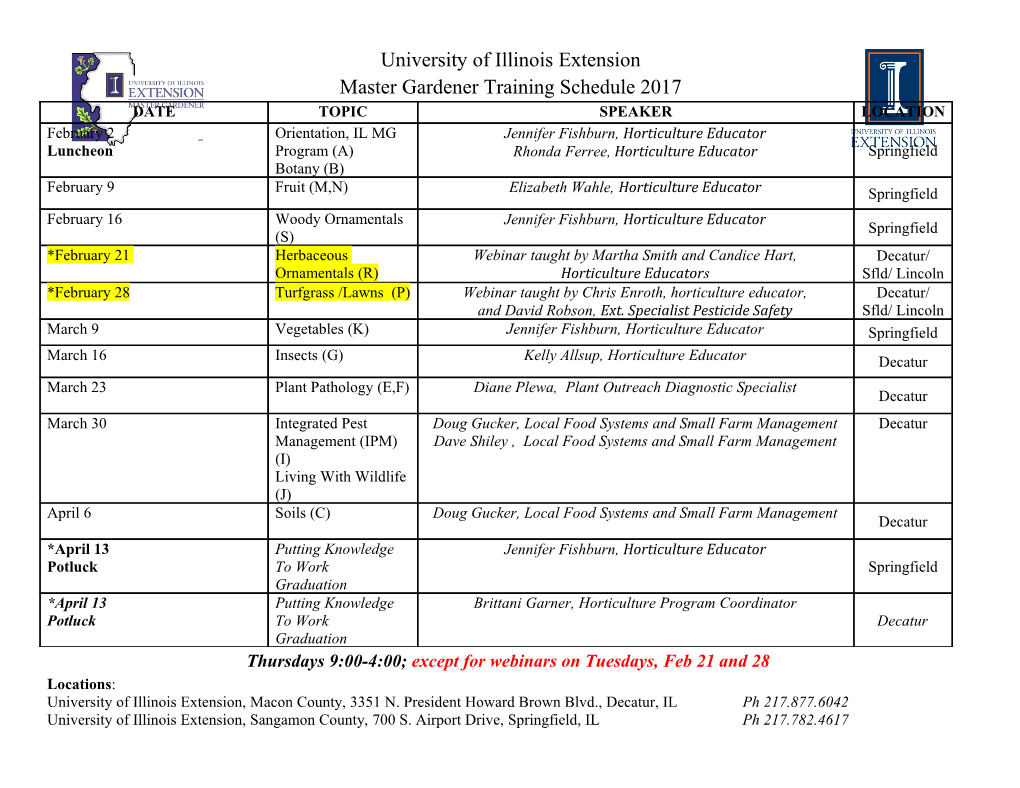
MECHANISTIC KINETIC MODELING OF THE HYDROCRACKING OF COMPLEX FEEDSTOCKS A Dissertation by HANS KUMAR Submitted to the Office of Graduate Studies of Texas A&M University in partial fulfillment of the requirements for the degree of DOCTOR OF PHILOSOPHY December 2006 Major Subject: Chemical Engineering MECHANISTIC KINETIC MODELING OF THE HYDROCRACKING OF COMPLEX FEEDSTOCKS A Dissertation by HANS KUMAR Submitted to the Office of Graduate Studies of Texas A&M University in partial fulfillment of the requirements for the degree of DOCTOR OF PHILOSOPHY Approved by: Co-Chairs of Committee, Gilbert F. Froment Rayford G. Anthony Committee Members, Perla B. Balbuena Abraham Clearfield Head of Department, N. K. Anand December 2006 Major Subject: Chemical Engineering iii ABSTRACT Mechanistic Kinetic Modeling of the Hydrocracking of Complex Feedstocks. (December 2006) Hans Kumar, B.E., Indian Institute of Technology, Roorkee; M.S., Texas A&M University, College Station. Co-Chairs of Advisory Committee: Dr. Gilbert F. Froment Dr. Rayford G. Anthony Two separate mechanistic kinetic models have been developed for the hydrocracking of complex feedstocks. The first model is targeted for the hydrocracking of vacuum gas oil. The second one addresses specifically the hydrocracking of long-chain paraffins, but at a more fundamental level as compared to the first one. Both models are based on an exhaustive computer generated reaction network of elementary steps. In the first model, the dehydrogenation/hydrogenation steps occurring on the metal sites to generate/consume the reactive olefinic intermediates are assumed to be very fast so that the acid site steps are considered as the rate determining steps. The frequency factors for acid site steps are modeled using the single-event concept and the activation energies based on the nature of the reactant and product carbenium ions. This model utilizes a detailed composition of the vacuum gas oil characterized by 16 different molecular classes up to carbon number 40. These classes are divided into 45 subclasses by distinguishing the isomers of a class according to the number of methyl branches. The kinetic model is plugged into an adiabatic multi-bed trickle flow reactor model. The model contains 33 feedstock and temperature independent parameters which have been estimated from the experimental data. The model has been used to study the effect of the operating conditions on the yield and composition of various products. A sensitivity analysis of the distribution of isomers of a iv class among its different subclasses has been performed showing that the total conversion increases when the content of isomers with a higher degree of branching is increased in the feed. In the second model, the dehydrogenation/hydrogenation steps on the metal sites are also assumed to be rate determining. The rate coefficients for the dehydrogenation steps are modeled depending on the nature of the carbon atoms forming the double bond. The frequency factors for the acid site steps are modeled using the single-event concept. A more rigorous approach has been selected to model the activation energies of the acid site steps by implementing the Evans-Polanyi relationship. The 14 model parameters, which are independent of the temperature and feedstock composition, have been estimated from the experimental data. The model elucidates the effect of the relative metal/acid activity of the catalyst on the isomerization/cracking selectivities and on the carbon number distribution of the products. v DEDICATION To my parents vi ACKNOWLEDGMENTS I would like to express my sincere gratitude to Dr. Gilbert F. Froment and Dr. Rayford G. Anthony for serving as the co-chairs of my committee and for their continued guidance and support in this research. The scientific discussions with Dr. Froment have always been very insightful and I will always be indebted to him for all the knowledge he shared with me. His prompt responses to all my email queries are truly appreciated. Dr. Anthony always assisted me with all the technical and non-technical issues during this research. His encouragement and interest led this project to be completed successfully in a timely manner. This research would not have been completed without the generosity of Dr. Anthony providing financial support from the C. D. Holland Professorship for this project. I am truly thankful to my advisors for the trust they showed in my capabilities to complete this research. I would also like to thank Dr. Perla Balbuena and Dr. Abraham Clearfield for being on my committee and for their time, efforts and ideas they contributed during our meetings. I am also grateful to Dr. R. E. Galiasso for various stimulating discussions and knowledge. My special thanks go to my friend and mentor, Dr. J. Govindhakannan. I had a wonderful time with him discussing different aspects of research and life. His previous contribution in this field is highly appreciated. I am also grateful to the faculty of the Department of Chemical Engineering for being extremely cooperative and friendly at all times during my stay at Texas A&M University. I would especially like to thank Dr. K. R. Hall, Dr. D. M. Ford, Dr. D. F. Shantz and Dr. M. El- Halwagi. The help that I received from our wonderful staff, especially Towanna Hubacek, Missy Newton, Valerie Green, Ninette Portales, Barbara Prout and Jeff Polasek, is simply unforgettable. Many thanks to my friends and my group members for making my research at Texas A&M University a pleasant and exciting experience. I am fortunate to have good friends vii like Arnab, Faisal, Amit, Vipin, Srini, Ashwini, Manish, Sanjay, Jyoti, Nishant, Hemendra, Greg and Nitin who were always there for me in good and bad times. Finally, I would like to express my warmest regards to my parents and family members for their unconditional love and support without which I could have never completed this dissertation. viii TABLE OF CONTENTS Page ABSTRACT..................................................................................................................... iii DEDICATION ...................................................................................................................v ACKNOWLEDGMENTS.................................................................................................vi TABLE OF CONTENTS ............................................................................................... viii LIST OF TABLES ............................................................................................................xi LIST OF FIGURES......................................................................................................... xii CHAPTER I INTRODUCTION ............................................................................................1 1.1. Hydrocracking Process Description...........................................................3 1.2. Literature Survey........................................................................................7 II HYDROCRACKING CHEMISTRY AND REACTION aaaaaaaaaa NETWORK GENERATION .........................................................................10 2.1. Hydrocracking Catalyst............................................................................10 2.2. Reaction Mechanism ................................................................................10 2.3. Reaction Network Generation..................................................................17 2.4. Composition of VGO ...............................................................................20 III KINETIC MODEL DEVELOPMENT FOR THE HYDROCRACKING aaaaaaaaai OF VGO ..........................................................................................................30 3.1. Modeling of the Frequency Factors .........................................................31 3.2. Modeling of the Activation Energies .......................................................34 3.3. Development of Rate Equations...............................................................36 3.3.1. Group (a)- PCP, Acyclic/Exo/Endo β-Scission.............................38 3.3.2. Group (b)-Cyclization ....................................................................41 3.3.3. Group (c)- Dealkylation of Aromatics ...........................................42 3.3.4. Saturation of Aromatics .................................................................43 3.3.4.1. Saturation of Monoaromatics ............................................43 3.3.4.2. Saturation of Polyaromatics ..............................................48 3.4. Competitive Chemisorption on the Metal Sites .......................................51 3.5. Development of Global Rate Expressions for Acid Site Steps ................52 3.6. Elimination of the Need of Reference Olefins .........................................59 3.6.1. Lumping Coefficients with Reference Olefins (Case 1)................60 3.6.2. Lumping Coefficients without Reference Olefins (Case 2)...........61 3.6.3. True Lumping Coefficients (Case 3)..............................................61 3.7. Competitive Chemisorption on the Acidic Sites......................................63 ix CHAPTER Page 3.7.1. Dehydrogenation Equilibrium Coefficient.....................................64 3.7.2. Heats of Protonation.......................................................................65 3.7.3. Heats of Stabilization.....................................................................68 3.7.4. Entropy of Protonation...................................................................69
Details
-
File Typepdf
-
Upload Time-
-
Content LanguagesEnglish
-
Upload UserAnonymous/Not logged-in
-
File Pages195 Page
-
File Size-