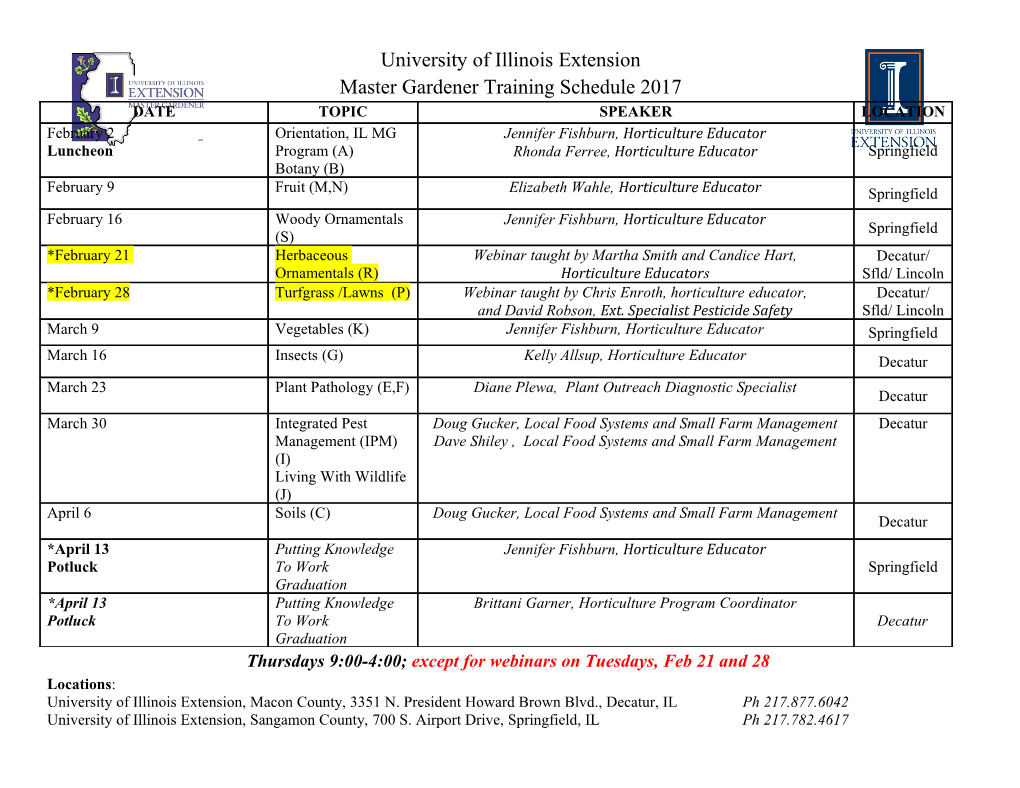
Hattoria 12: 9–25. 2021 Evolutionary leverage of dissilient genera of Pleuroweisieae (Pottiaceae) evaluated with Shannon-Turing analysis Richard H. ZANDER Missouri Botanical Garden, 4344 Shaw Blvd., St. Louis, Missouri 63116, U.S.A. Author for correspondence: Richard H. ZANDER, [email protected] Abstract Evolutionary leverage is the average of total transformational trait changes among species in a dissilient (radiative) genus or larger, integral taxonomic group. It is proposed as a measure of evolutionary success in modern environments. Comparing evolutionary leverage aids in measuring potential evolutionary coherency and ecological success among taxonomic groups, here exemplified with Pleuroweisieae and Barbuloideae of Pottiaceae and genera of Streptotrichaceae (all Bryophyta). Shannon-Turing analysis—calculation using summed informational bits and sequential Bayes— translates to relative support for order and coherence of species in dissilient (radiative) genera. Hymenostylium gracillimum (Pottiaceae) is transferred to Ardeuma, while H. xerophilum and H. hildebrandtii (Merceyopsis hymenostylioides as synonym) are transferred to Eobryum. These three possess the primitive trait of a stem central strand in Pleuroweisieae. The genus Ozobryum is resurrected from synonymy with Molendoa. Introduction “Evolutionary leverage” is a term used for suites of adaptations that presumably allow better competition against other species, e.g. angiosperm success over competing groups (Brodribb & Feild 2010). Evolutionary leverage is more exactly defined here as a comparison of the average number of trait transformations among newly generated species in a dissilient genus as a closed causal group (Zander 2018: 36). A dissilient genus is one characterized by a progenitor species with apparent descendant species radiating from it without reversals in key traits (reversed from those in an outgroup or the inferred progenitor of a progenitor) or at least very few reversals. When such a radiation is evident, a phylogenetically monophyletic group may be divided into smaller, empirically distinguishable genera. A closed causal group is one for which the species are indisputably a member of that group, and probabilistic evaluations of taxon order of speciation may safely ignore the possibility of membership in other groups. That is, evolutionary leverage is measured by the average number of new traits in a speciation event summed over all species in a genus in one well-characterized radiation—the traits being either adaptive or nearly neutral (e.g. from drift). 9 It is expected that many large genera require taxonomic dissection into smaller, more coherent genera that exhibit few if any reversals in major traits within a genus during speciation events. Trait changes when they occur in spite of stabilizing and purifying selection are scattered apparently randomly among many possible traits (in Pottiaceae more than 70, Zander 1993). Reversals in apparently neutral traits that have been proved to be taxonomically important for one species are revealed as taxonomically unimportant at the genus level by other conservative traits. The fact that this methodology provides evolutionary relationships that are parsimonious, matches fairly well with molecular systematics, and fits evolutionary theory is an existence proof of this variant of Dollo parsimony, and argues for a general retention of taxonomically important evolutionarily conservative traits over millions of years rather than among only a few living fossils. Gould (1970) has additional arguments in support of minimizing trait reversals in evolutionary analysis. Such dissilient (radiating) genera are mostly without changes in shared conservative, ancestral traits. The average number of trait changes in species in a genus, that is from progenitor to descendant, is an integration of two important values, the number of species in a genus, and the number of new traits associated with a speciation event in each of those species. Such new traits are commonly those used in a standard key distinguishing species. The total trait transformations and the total number of species are measures of evolutionary success in modern environments, as (1) multiple adaptations around a single set of fundamental traits, (2) a single combination of basal traits sufficiently robust as to tolerate a number of somewhat encumbering anagenetic mutations gradually accumulated among descendant species, or (3) some combination of these. High evolutionary leverage signals demonstrated evolvability, that is, the ability to respond to selective pressure. In the context of an ecosystem, large genera with well adapted traits are an empirically defined taxonomic group above that of species. Expressed as an average number of trait changes per species in a genus, evolutionary leverage reflects a degree of demonstrated persistence among competing species and changing environments. This measure works well with genera conceived as centers of radiation or dissilience, i.e., a putative progenitor species and its descendants. The rationale for naming ancestors is that if a modern species has the traits inferred for an ancestor, then that ancestor can be in practice named the same. Most of the presently recognized large genera need translation into smaller dissilient genera, and some genera may have poorly distinguished species (few trait transformations) while others have many. Past analyses of genera using principles of macroevolutionary systematics (see Zander 2019c) have pointed out that when species of a genus fit together well, particularly if generated with no reversals of important traits, then the whole genus has a better chance of modeling an integral ecological unit in evolution. The average number of trait transformations per species in a dissilient genus measures the degree of morphological heterogeneity between species. A “good” genus is one with an expected number of traits per species, such as that within two standard deviations of the number of traits per species evidenced among related dissilient genera. Past studies have shown that there are about four trait transformations on average from progenitor to descendant in taxa of the moss family Pottiaceae. Although many transformations per speciation event are possible (some punctuational, some perhaps anagenetic), retention of the immediate ancestral morphotype confirms membership in the 10 genus as a closed causal group. Easy determination of first, second, third and fourth standard deviations (sigmas) is available to taxonomists via the Running Sigmas Calculator (Zander 2020), an Microsoft Excel spreadsheet available online. Materials and Methods Background Because macroevolutionary systematics is a new technique for generating evolution- based classifications, a summary of principles and protocols is given here distilled from more expansive presentations by Zander (2013, 2018, 2019a, 2019b, 2019c). The object is to generate from expressed traits apparently subject to adaptive or nearly neutral fixation a model parsimoniously arranging morphological trait transformations associated with speciation between sequential pairs of species. The idea is simple. Starting with a group of previously selected related species, select three closely related species and attach them serially as a (1) putative outgroup or prior ancestor, (2) a progenitor, and (3) a descendant such that there are no (or minimum) reversals of the descendant back to the outgroup or prior ancestor. Continue inserting additional species at the beginning, middle or end of this series, allowing branching when series end. This is like the construction of a “Besseyan cactus” but is composed of species. A set of diagrams illustrating this process is given by Zander (2018: 81). The requirement of no (or minimal) reversals makes this a second order Markov chain where the last element partially depends on the antepenultimate of any serial set of three species. The antepenultimate is always an outgroup for the next two species in a lineage. Arranging an evolutionary tree such that there are no reversals also models the maximum preservation of the number of conservative traits through the speciation process. The diagram of this model is a speciation tree, or caulogram. This focuses on transformations between species, dealing with each species pair, one by one. A trait involved in speciation is one commonly used in a standard key to species. Optimization is by arrangement of species such that there are no or few trait reversals in a lineage. Because standard preliminary taxonomic analysis reduces the number of species involved to very few, massive computerized analysis is generally unnecessary. Phylogenetics, on the other hand, develops dichotomous non-ultrametric dendrograms, or cladograms, based on optimized dichotomous transformations between sequentially dichotomously split sets of traits. Macroevolutionary systematics, conversely, models the inferred order of speciation between the individual elements of a lineage, that is, between two species in the context of an outgroup or inferred ancestor. Phylogenetics searches for a pattern with maximum likelihood or maximum posterior probability (Felsenstein 2004: 256, 291) of the shared ancestry of sets of whole set of sampled taxa at once. The optimum pattern stands out from a distribution curve as an outlier. Support for caulograms is by posterior probabilities of a putative ancestral species generating a descendant species given a particular outgroup or accepted shared ancestor.
Details
-
File Typepdf
-
Upload Time-
-
Content LanguagesEnglish
-
Upload UserAnonymous/Not logged-in
-
File Pages17 Page
-
File Size-