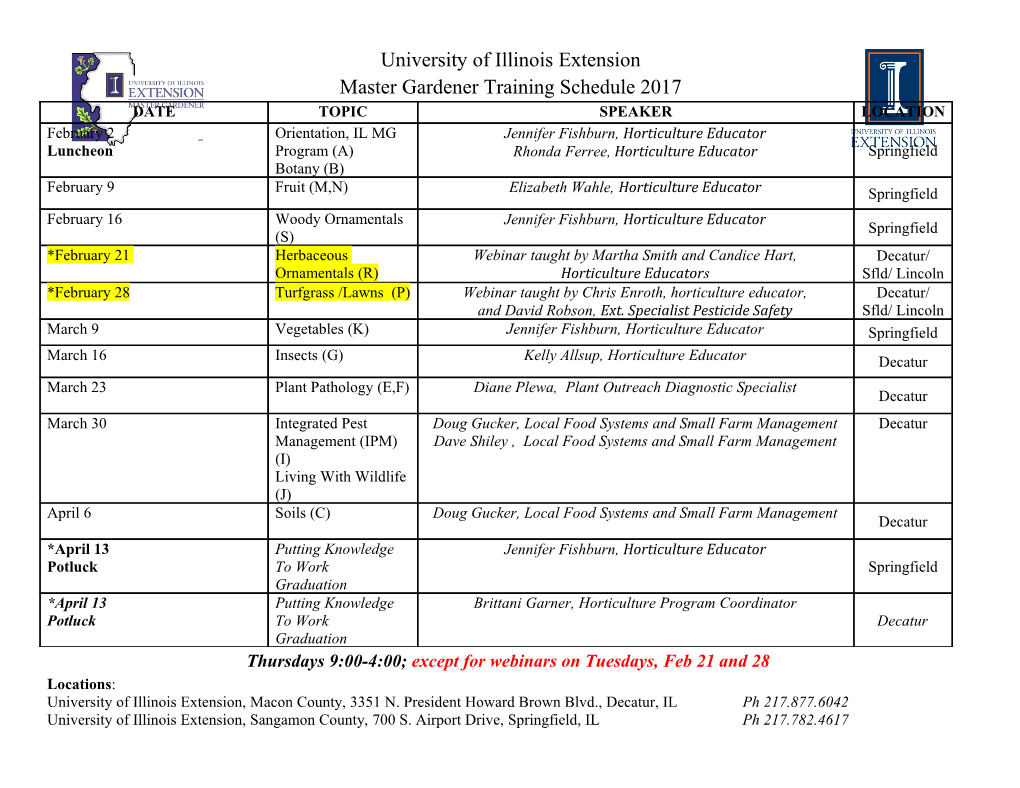
applied sciences Article In-Situ Photo-Dissociation and Polymerization of Carbon Disulfide with Vacuum Ultraviolet Microplasma Flat Lamp for Organic Thin Films Jinhong Kim 1 and Sung-Jin Park 1,2,* 1 Laboratory of Optical Physics and Engineering, Department of Electrical and Computer Engineering, University of Illinois at Urbana-Champaign, Urbana, IL 61801, USA; [email protected] 2 Eden Park Illumination, Inc., Champaign, IL 61821, USA * Correspondence: [email protected] Abstract: Vacuum UV (VUV) photo-dissociation for a liquid phase organic compound, carbon disulfide (CS2), has been investigated. 172 nm (7.2 eV) VUV photons from Xe2* excimers in a microcavity plasma lamp irradiated free-standing liquid droplets on Si substrate in each a nitrogen environment and an atmospheric air environment. Selective and rapid dissociation of CS2 into C-C, C-S or C-O-S based fragments was observed in the different gas environments during the reaction. Thin-layered polymeric microdeposites have been identified by characterization with a Scanning electron microscope (SEM), Energy dispersive x-ray spectroscopy (EDX), Raman spectroscopy and X-ray photoelectron spectroscopy (XPS). This novel photo-process from the flat VUV microplasma lamp introduces another pathway of low-temperature organic (or synthetic) conversion for large area deposition. The in-situ, selective conversion of various organic precursors can be potentially used in optoelectronics and nanotechnology applications. Citation: Kim, J.; Park, S.-J. In-Situ Keywords: carbon disulfide; microplasma; photo-dissociation; polymerization; 172 nm VUV lamp Photo-Dissociation and Polymerization of Carbon Disulfide with Vacuum Ultraviolet Microplasma Flat Lamp for Organic Thin Films. Appl. Sci. 2021, 11, 2597. 1. Introduction https://doi.org/10.3390/app11062597 Low temperature deposition techniques are becoming essential for many types of opto- and microelectronics fabricated in flexible substrates. Several approaches are currently Academic Editor: Andrea Li Bassi being utilized to avoid damage on fragile substrates, such as plasma-enhanced chemical deposition (PECVD) and laser chemical deposition (LCVD) [1,2]. However, malleable Received: 18 February 2021 substrates are subjected to high energetic ionic bombardment caused by plasma generation Accepted: 12 March 2021 in the PECVD system, producing cracking and unwanted stoichiometry ratios in the Published: 15 March 2021 deposited material [3–5]. In an LCVD system, lasers are costly and provide only a small area deposition about several cm2. Alternatively, an incoherent UV source can be used Publisher’s Note: MDPI stays neutral in many processing applications, since most organic/inorganic materials readily absorb with regard to jurisdictional claims in photons in wavelengths < ~250 nm. However, the utilization of traditional UV sources published maps and institutional affil- (UVC) in chemical dissociation is still limited by insufficient photon energy associated with iations. reactants’ chemical bonds, and ends in slow process rates (kinetics) unless it uses photo- catalysts. A new VUV (172 nm) flat lamp [6] has distinct benefits such as high radiation power, large and uniform area coverage that was not possible in the conventional photon sources for chemical reactions. In this research article, the experiment for dissociation Copyright: © 2021 by the authors. of CS2 by a VUV lamp will be described in the different experimental conditions. As a Licensee MDPI, Basel, Switzerland. conductive polymer, CS2 has been used as a sulfur dopant in organic chemistry and a This article is an open access article solvent for various polymers [7,8]. In addition, CS2 can be used as an etchant of the carbon distributed under the terms and layer when it is used with plasma [9]. It has been known that CS2 can be readily dissociated conditions of the Creative Commons to CS + S fragments through photons, catalytic reactions, and plasma processes in the Attribution (CC BY) license (https:// vapor phases [10–12]. However, direct photodissociation of a free standing and volatile creativecommons.org/licenses/by/ CS droplets on a substrate using high energy VUV photons (>7.2 eV) to deposit a thin 4.0/). 2 Appl. Sci. 2021, 11, 2597. https://doi.org/10.3390/app11062597 https://www.mdpi.com/journal/applsci Appl. Sci. 2021, 11, x FOR PEER REVIEW 2 of 9 in the vapor phases [10–12]. However, direct photodissociation of a free standing and vol- Appl. Sci. 2021, 11, 2597 atile CS2 droplets on a substrate using high energy VUV photons (>7.2 eV)2 to of 10deposit a thin layer that has never been explored. In addition, the conversion of the photo-fragments to a thin layer deposit in a scaled area of Si substrate is introduced. Being the first report of its kind for an in-situ high energy UV deposition methodology, the low temperature layer that has never been explored. In addition, the conversion of the photo-fragments to anda thin simple layer depositdeposition in a scaledtechnology area of of Si organic substrate precursors is introduced. will Being lead theto the first cost-effectiveness report of ofits microelectronics kind for an in-situ processes. high energy UV deposition methodology, the low temperature and simple deposition technology of organic precursors will lead to the cost-effectiveness of 2.microelectronics Experimental processes. Method 2. ExperimentalThe operation Method principle of excimer lamps relies on the radiative decomposition of excimer dimers such as Xe2* (172 nm) formed in a plasma discharge of noble gases. As The operation principle of excimer lamps relies on the radiative decomposition of illustrated in Figure 1a, Xe2 is well known for emitting bright continua (150, 172 nm) along excimer dimers such as Xe2* (172 nm) formed in a plasma discharge of noble gases. As with resonance lines of Xe* (129 and 147 nm). The dominant Xe2 emission at 172 nm arises illustrated in Figure1a, Xe 2 is well known for emitting bright continua (150, 172 nm) along 1 1 fromwithresonance A ∑ → linesX ∑ of transitions Xe* (129 and of 147 the nm). molecule The dominant and its Xe emission2 emission intensities’ at 172 nm arises growth are 1 + 1 + attributedfrom A ∑u to! theX ∑ three-bodyg transitions collision of the molecule of excited and state its emission Xe* and intensities’ 2Xe ground growth state are atoms [6]. Theattributed formation to the rate three-body of these collision three ofbody excited reactions state Xe* is andincreased 2Xe ground quadratically state atoms with [6]. Xe gas pressure.The formation The ratestructure of these of threethe microplasma body reactions lamp is increased used here quadratically has an array with of Xe microcavities gas thatpressure. produces The structure a low temperature of the microplasma and non-equi lamp usedlibrium here plasmas. has an array The of electron microcavities temperature that produces a low temperature and non-equilibrium plasmas. The electron temperature of microplasma lies in the 2–5 eV interval, which is ideal for forming the excimer (Xe2) and of microplasma lies in the 2–5 eV interval, which is ideal for forming the excimer (Xe2) increasingand increasing its transition its transition intensities. intensities. Another Another advantageadvantage of of using using excimer excimer lamps lamps is that is that the non-stablethe non-stable ground ground state state of ofan an excimer excimer lamp lamp is is able able to have nono self-absorptionself-absorption of of the the emit- tedemitted illumination, illumination, providing providing a ahigh high UV UV intensity. intensity. InInaddition, addition, the the flat flat form form factor factor and and dif- fusivediffusive glow glow in in the the lamp lamp makemake a a uniform uniform treatment treatment over over thearea the ofarea the of lamp. the Therefore,lamp. Therefore, thethe depositiondeposition area area can can be be easily easily scaled scaled along along with with the flat the lamp flat sizelamp at size given at intensities. given intensities. Figure 1.1.( a(a)) Basic Basic energy energy and and transition transition diagram diagram of VUV of emission VUV emission of Xe2 excimer of Xe2 lamp, excimer (b) Schematic lamp, (b) Sche- maticpotential potential energy curveenergy of thecurve target of molecule,the target CS molecule,2 with 172 CS nm2 VUVwith lamp 172 nm energy VUV level lamp (red energy dash line). level (red dash line). Appl. Sci. 2021, 11, 2597 3 of 10 Appl. Sci. 2021, 11, x FOR PEER REVIEW 3 of 9 Figure2a demonstrates the operation of the 172 nm microplasma VUV lamp (100 × 100 mm, Eden Park Illumination, Inc. Champaign, IL, USA). The lamp is filled with 500 Torr of 70% Xe/Ne gas mixture, and operated at 20–30 kHz of 3 kV pulses. The outputFigure intensity 2a demonstrates at the surface the operation of the lamp of the was 172 17nm mW/cm microplasma2. Figure VUV2 lampb shows (100 the× VUV emission100 mm, Eden spectrum Park Illumination from the lamp., Inc. FigureChampaign,1b is aIL, schematic USA). The of lamp the potentialis filled with energy 500 curves Torr of 70% Xe/Ne gas mixture, and operated at 20–30 kHz of 3 kV pulses. The output in the excited states of CS [13]. The red dashed line corresponds to the 172 nm VUV lamp intensity at the surface of the2 lamp was 17 mW/cm2. Figure 2b shows the VUV emission photon energy (7.2 eV) in this experiment. Photon energy from the VUV lamp sufficiently spectrum from the lamp. Figure 1b is a schematic of the potential energy curves in the covers the energies required to dissociate the C=S bond of the CS . The configuration of excited states of CS2 [13]. The red dashed line corresponds to the 172 nm 2VUV lamp pho- theton VUVenergy photodissociation (7.2 eV) in this experiment. setup were Phot shownon energy in Figure from2 c.the The VUV distance lamp sufficiently between the VUV lampcovers and the substrateenergies required was maintained to dissociate 1 the cm.
Details
-
File Typepdf
-
Upload Time-
-
Content LanguagesEnglish
-
Upload UserAnonymous/Not logged-in
-
File Pages10 Page
-
File Size-