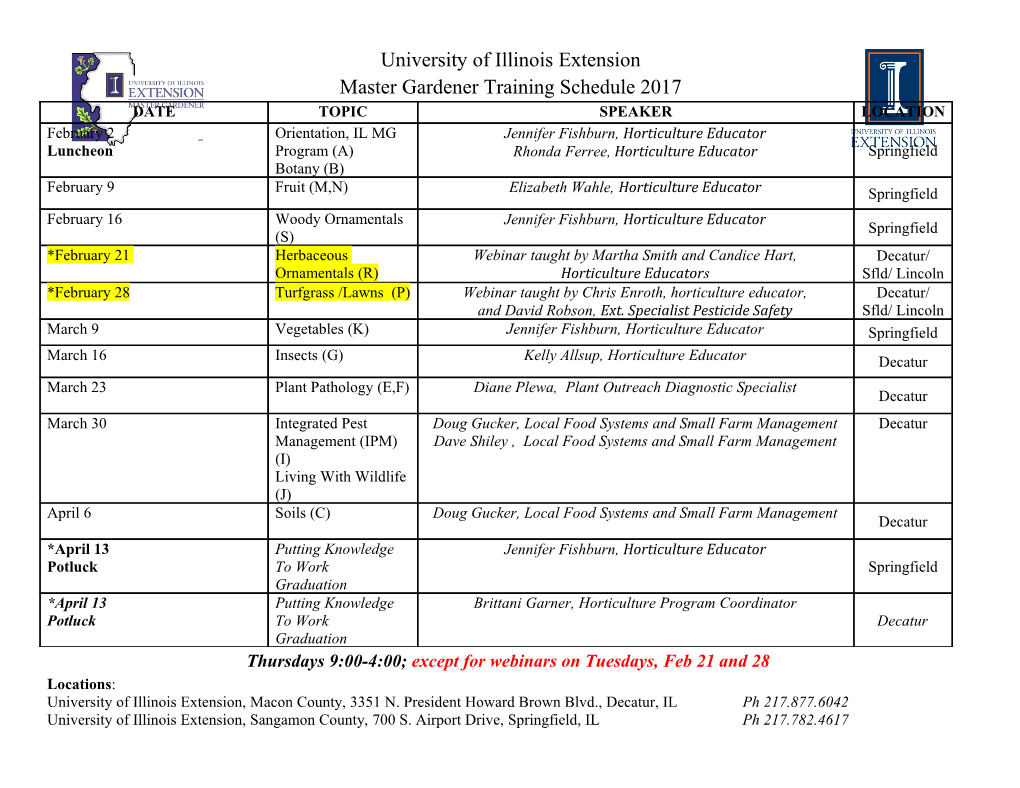
Rupture process of the Mw=7.9 2015 Gorkha earthquake (Nepal): insights into Himalayan megathrust segmentation Raphaël Grandin, Martin Vallée, Claudio Satriano, Robin Lacassin, Yann Klinger, Martine Simoes, Laurent Bollinger To cite this version: Raphaël Grandin, Martin Vallée, Claudio Satriano, Robin Lacassin, Yann Klinger, et al.. Rupture process of the Mw=7.9 2015 Gorkha earthquake (Nepal): insights into Himalayan megathrust seg- mentation. Geophysical Research Letters, American Geophysical Union, 2015, 42 (20), pp.8373-8382. 10.1002/2015GL066044. hal-01214961v2 HAL Id: hal-01214961 https://hal.archives-ouvertes.fr/hal-01214961v2 Submitted on 24 Oct 2017 HAL is a multi-disciplinary open access L’archive ouverte pluridisciplinaire HAL, est archive for the deposit and dissemination of sci- destinée au dépôt et à la diffusion de documents entific research documents, whether they are pub- scientifiques de niveau recherche, publiés ou non, lished or not. The documents may come from émanant des établissements d’enseignement et de teaching and research institutions in France or recherche français ou étrangers, des laboratoires abroad, or from public or private research centers. publics ou privés. Copyright PUBLICATIONS Geophysical Research Letters RESEARCH LETTER Rupture process of the Mw =7.9 2015 Gorkha 10.1002/2015GL066044 earthquake (Nepal): Insights into Himalayan Key Points: megathrust segmentation • Uniform rupture velocity during peak moment release explains modest Raphaël Grandin1, Martin Vallée1, Claudio Satriano1, Robin Lacassin1, Yann Klinger1, acceleration in Kathmandu 1 2 • High-frequency radiation occurred Martine Simoes , and Laurent Bollinger preferentially along the downdip 1 2 edge of the high slip patch Institut de Physique du Globe de Paris, Sorbonne Paris Cité, Université Paris Diderot, CNRS, Paris, France, CEA, DAM, DIF, • The MHT is locked updip of the 2015 Arpajon, France event, suggesting high level of seismic hazard toward the front Abstract We investigate the rupture process of the 25 April 2015 Gorkha earthquake (Mw = 7.9) using a Supporting Information: kinematic joint inversion of teleseismic waves, strong motion data, high-rate GPS, static GPS, and synthetic • Data Set S1 aperture radar (SAR) data. The rupture is found to be simple in terms of coseismic slip and even more in terms • Data Set S2 of rupture velocity, as both inversion results and a complementing back projection analysis show that the • Data Set S3 – • Data Set S4 main slip patch broke unilaterally at a steady velocity of 3.1 3.3 km/s. This feature likely contributes to the • Figures S1–S9, Tables S1 and S2, and moderate peak ground acceleration (0.2 g) observed in Kathmandu. The ~15 km deep rupture occurs along Captions for Data Sets S1–S4 the base of the coupled portion of the Main Himalayan Thrust and does not break the area ranging from Kathmandu to the front. The limitation in length and width of the rupture cannot be identified in the Correspondence to: R. Grandin, preearthquake interseismic coupling distribution and is therefore discussed in light of the structural [email protected] architecture of the megathrust. Citation: Grandin, R., M. Vallée, C. Satriano, 1. Introduction R. Lacassin, Y. Klinger, M. Simoes, and L. Bollinger (2015), Rupture process of Central Nepal, including its capital Kathmandu, has been considerably damaged by the Gorkha earthquake the Mw = 7.9 2015 Gorkha earthquake of 25 April 2015. The event resulted in more than 9000 fatalities, mostly due to building collapse and (Nepal): Insights into Himalayan megathrust segmentation, Geophys. Res. induced landslides. Tectonically, the Gorkha earthquake is rooted in the core of the Main Himalayan Lett., 42,8373–8382, doi:10.1002/ Thrust (MHT) system, which accommodates the 18 mm/yr convergence between India and Tibet [Avouac 2015GL066044. and Tapponnier, 1993; Lavé and Avouac, 2000] (Figure 1). Geology, thermochronology, and geomorphology suggest that the plate interface hosts several flats and ramps [e.g., Bollinger et al., 2004b; Herman et al., Received 3 SEP 2015 Accepted 8 OCT 2015 2010], which makes the MHT more geometrically complex than most subduction megathrusts. However, Accepted article online 12 OCT 2015 geodetic and seismological studies indicate that the ~100 km most frontal portion of the MHT was fully Published online 24 OCT 2015 locked and largely aseismic in the decades preceding the Gorkha earthquake. Therefore, the actual geome- try of the MHT in the shallow seismogenic zone is difficult to determine from geodesy or seismology [e.g., Vergne et al., 2001]. Historical seismicity associated with the MHT is characterized by infrequent, large megathrust earthquakes that occasionally break the MHT up to its frontal expression along the Main Frontal Thrust (MFT) [Bilham et al., 2001]. Paleoseismology has provided evidence for earthquakes with magnitudes exceeding M8.5 asso- ciated with surface ruptures reaching the MFT [e.g., Sapkota et al., 2013] (Figure 1). However, historical records suggest that large, blind earthquakes do also take place, such as the 1833 earthquake (M7.3–7.7) that occurred near Kathmandu [Szeliga et al., 2010]. The significance of these blind earthquakes in releasing part of the interseismically accumulated strain is of paramount importance for quantifying slip deficit of the MHT and for assessing associated seismic hazard. The 2015 Mw 7.9 Gorkha earthquake is the largest earthquake occurring on the MHT since the great 1934 Bilhar-Nepal earthquake [Sapkota et al., 2013]. As the MHT appears to remain fully locked along most of its length [Ader et al., 2012], the Gorkha earthquake provides the first opportunity to bring to light the seismo- genic characteristics of the MHT. Using a combination of seismological and geodetic observations, we jointly constrain the static and kinematic characteristics of the Gorkha earthquake rupture. The features of the rupture model are related ©2015. American Geophysical Union. to the local seismic radiations recorded in Kathmandu and interpreted in the light of the broader context All Rights Reserved. of preseismic strain accumulation and long-term architecture of the MHT. GRANDIN ET AL. RUPTURE PROCESS OF THE GORKHA EARTHQUAKE 8373 Geophysical Research Letters 10.1002/2015GL066044 Figure 1. Tectonic and paleoseismological background of the 2015 Gorkha earthquake. Thick dashed arrows indicate lateral extension of surface rupture of past great earthquakes. Location of the 1833 earthquake (in blue) is from Bollinger et al. [2015]. The pink and brown dotted lines show the area of significant slip (>2 m) of the 25 April 2015 Gorkha earth- quake main shock and the 12 May 2015 aftershock, respectively (this study). Epicenter of the main shock reported by NSC is indicated by the pink star. Green and purple dashed lines show the location and along-strike tapering of the Pokhara-Gorkha anticline and Kathmandu synclinal, respectively. Thick grey lines delimit the inferred Kathmandu segment. The 1000 m and 3000 m elevation contours are represented by thin black lines. White triangles indicate peaks with elevation higher than 8000 m. Normal faults are indicated by grey barbed lines. MFT, Main Frontal Thrust; MBT, Main Boundary Thrust; MCT, Main Central Thrust; and K, Kathmandu. 2. Data and Methods 2.1. Broadband Kinematic Inversion of the Rupture Process The Gorkha earthquake has been recorded by different types of geodetic and seismic sensors, including synthetic aperture radar (SAR) and continuous GPS measurements, local ground motion, and teleseismic data. For this large shallow continental earthquake, SAR data are particularly valuable to constrain the spatial distribution of final slip, while ground motion data control its temporal evolution. The line-of-sight (LOS) component of static surface displacement is mapped using SAR data acquired by the C band satellite Sentinel-1A of the European Space Agency and the L band satellite ALOS-2 of the Japan Aerospace Agency (Table S1 in the supporting information). Both ascending and descending geometries are used to constrain separately horizontal and vertical components of ground motion (Figure 2c). The short revisit time of the two platforms made it possible to isolate the coseismic displacement induced by the 12 May 2015 large aftershock (Mw 7.3). Sentinel-1 data are processed using the interferometric SAR (InSAR) technique according to the method of Grandin [2015]. Unfortunately, interferometric coherence was insuffi- cient to ensure correct phase unwrapping for the main shock, due to the combination of large surface displa- cements, rough topography, vegetation and snow/ice cover, large perpendicular baselines, and possibly pervasive landsliding. Alternatively, dense pixel tracking was computed, taking advantage of the fine range resolution of the platform (2.3 m), yielding a theoretical accuracy of the order of 20 cm in the LOS. The ALOS-2 InSAR data are from Lindsey et al. [2015]. GRANDIN ET AL. RUPTURE PROCESS OF THE GORKHA EARTHQUAKE 8374 Geophysical Research Letters 10.1002/2015GL066044 Figure 2. Rupture process of the Gorkha earthquake. (a) Slip distribution with 2 m slip contours. Grey dashed line deline- ates the surface projection of the modeled fault. Grey arrows show the direction of slip. Yellow symbols are back projection peaks, with their size proportional to the relative stack power. Grey circles are M > 4.5 aftershocks reported by Adhikari et al. [2015] until 8 June 2015. White star is NSC hypocenter. White square indicates location of Kathmandu city. Red symbols show the location of the closest stations used in this study. Slip model of the 12 May aftershock constrained by inversion of InSAR data (Figure S8) is indicated by a dashed contour. (b) Snapshots of slip distribution as a function of time deduced from kinematic inversion. Squares and circles indicate location of back projection peaks for every time frame. (c) Observed and modeled surface displacement from ALOS-2 descending InSAR (left column) and Sentinel-1 ascending range pixel tracking (right column). The black arrow indicates line-of-sight (LOS) direction. A positive sign indicates motion toward the satellite, whereas negative sign corresponds to motion away from the satellite.
Details
-
File Typepdf
-
Upload Time-
-
Content LanguagesEnglish
-
Upload UserAnonymous/Not logged-in
-
File Pages11 Page
-
File Size-