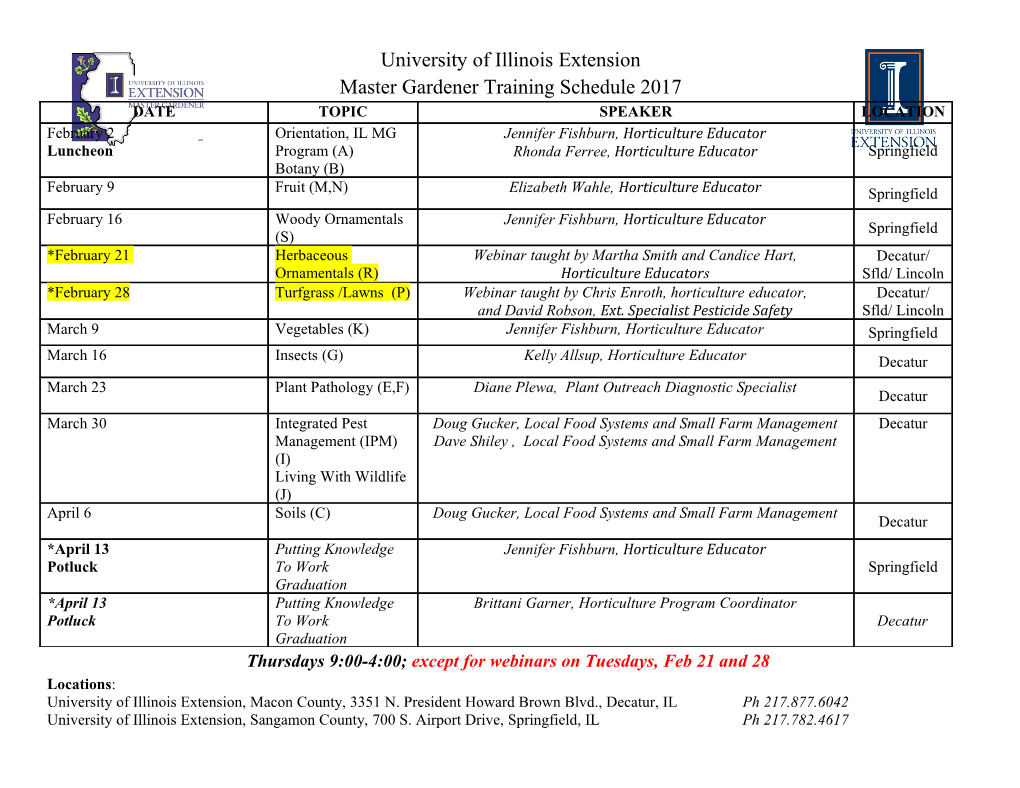
Confidential manuscript submitted to Reviews of Geophysics The Antarctic Slope Current in a Changing Climate Andrew F. Thompson1, Andrew L. Stewart2, Paul Spence3and Karen J. Heywood4 1Environmental Sciences and Engineering, California Institute of Technology, Pasadena, California, USA 2Department of Atmospheric and Oceanic Sciences, University of California, Los Angeles, California, USA 3Climate Change Research Center, University of New South Wales, Sydney, Australia 4Centre for Ocean and Atmospheric sciences, School of Environmental Sciences, University of East Anglia, UK Key Points: • Dynamical properties of the Antarctic Slope Current (ASC) that impact heat trans- port are reviewed. • A geographical classification of the ASC’s frontal structure is presented. • The potential for feedbacks between the ASC circulation and larger-scale climate is summarized. Corresponding author: Andrew F. Thompson, [email protected] –1– Confidential manuscript submitted to Reviews of Geophysics Abstract The Antarctic Slope Current (ASC) is a coherent circulation feature that rings the Antarctic continental shelf and regulates the flow of water towards the Antarctic coast- line. The structure and variability of the ASC influences key processes near the Antarctic coastline that have global implications, such as the melting of Antarctic ice shelves and water mass formation that determines the strength of the global overturning circulation. Recent theoretical, modeling, and observational advances have revealed new dynamical properties of the ASC, making it timely to review. Earlier reviews of the ASC focused largely on local classifications of water properties of the ASC’s primary front. Here, we instead provide a classification of the current’s frontal structure based on the dynamical mechanisms that govern both the along-slope and cross-slope circulation; these two modes of circulation are strongly coupled, similar to the Antarctic Circumpolar Current. Highly variable motions, such as dense overflows, tides, and eddies are shown to be critical com- ponents of cross-slope and cross-shelf exchange, but understanding of how the distribution and intensity of these processes will evolve in a changing climate remains poor due to ob- servational and modeling limitations. Results linking the ASC to larger modes of climate variability, such as El Niño, show that the ASC is an integral part of global climate. An improved dynamical understanding of the ASC is still needed to accurately model and pre- dict future Antarctic sea ice extent, the stability of the Antarctic ice sheets, and the South- ern Ocean’s contribution to the global carbon cycle. 1 Introduction Ocean heat transport and other oceanic processes occurring along the Antarctic coastline are vital to Earth’s climate. By influencing the oceanic flow towards the Antarc- tic continent, the Antarctic Slope Current (ASC), a coherent westward circulation and frontal feature that encircles the Antarctic continental shelf, is the linkage between these processes and the rest of the global ocean [Jacobs, 1991] (Fig. 1). The intensity and vari- ability of the ASC controls the rate at which heat moves across the continental slope and on to the continental shelf. Eventually, the circulation over the continental shelf brings this warm water into contact with marine-terminating glaciers of the Antarctic Ice Sheet (AIS). The ASC is a unique feature that responds not only to local changes, for example the surface wind stress [Gill, 1973; Stewart and Thompson, 2015a], but also to larger-scale modes of climate variability, such as El Niño and the Southern Annular Mode [Dutrieux et al., 2014; Spence et al., 2014a; Nakayama et al., 2018]. Shifts in the intensity or posi- tion of ASC fronts, or changes to the range of densities that occupy the continental shelf, will therefore exert a strong influence over the heat budget of the Antarctic margins. Increases in the heat content of Antarctic shelf waters [Schmidtko et al., 2014; Hey- wood et al., 2014] have contributed to changes in the AIS, especially in West Antarctica. One of the most dramatic signals has been a large-scale thinning of floating Antarctic ice shelves over most of the West Antarctic Ice Sheet (WAIS) [Cook and Vaughan, 2010; Paolo et al., 2015]. Most of the ice shelf thinning has been attributed to melting from be- low due to the circulation of warm ocean water coming into contact with the floating ice shelves [Pritchard et al., 2012]. This thinning has been implicated in the observed retreat of the grounding line of certain WAIS glaciers [Rignot et al., 2014] as well as the acceler- ation of ice sheet flow of WAIS glaciers [Joughin et al., 2002]. Enhanced basal melt rates due to warm ocean waters have also been observed in regions of East Antarctica, for ex- ample at Totten Glacier [Rintoul et al., 2016]. Melting by the ocean has contributed to an acceleration in the rate of ice loss of the WAIS; the rate of loss between 2012 and 2017, 159 ± 26 billion tonnes per year, was more than three times the rates estimated in 1990’s [IMBIE team, 2018]. These changes motivate the need for continued observations and improved mech- anistic understanding of physical processes that control the flow of heat across the ASC –2– Confidential manuscript submitted to Reviews of Geophysics Figure 1. Sketch of the along- and cross-slope circulations associated with the Antarctic Slope Current (ASC) system. Schematics of the ASC on either side the Antarctic Peninsula illustrate regional differences in the ASC. The left-hand panel shows key processes that occur in the Weddell Sea. Here, dense water forma- tion on the shelf is exported via bottom water outflows, enabling an onshore transport of warm Circumpolar Deep Water (CDW) that is isolated from frictional surface and bottom boundary layers. Over much of West Antarctica, for instance in the Bellingshausen Sea (right-hand panel), where bottom water formation is absent, CDW floods the upper continental slope and shelf. Strong along-slope winds in East Antarctica and the Wed- dell Sea maintain a strong shelf break front; surface winds are less uniform in West Antarctica. Topographic steering of the ASC at major bathymetric troughs is a prominent feature. Classifications of regional variations in the ASC is discussed in more detail in §2. Note that the vertical scale in this figure has been distorted to emphasize ocean processes and circulation. and towards the AIS. Quantifying how the ASC has changed over time, and how it might evolve in the future, is a challenging goal as waters responsible for the thinning of Antarc- tic ice shelves reside below the surface. This review seeks to provide an updated perspec- tive on the spatial and temporal variability of the ASC and its place in the larger climate system; we also highlight key areas for future research. 1.1 ASC characteristics The ASC is one of the ocean’s most extensive and coherent current systems (Fig. 2), sustaining along-slope velocities of 10-30 cm s−1 over a span of 21,000 km on Antarc- tica’s upper continental slope. Yet it remains remarkably understudied compared to other major oceanic circulation features, such as western boundary currents, or the Southern Ocean’s Antarctic Circumpolar Current (ACC). The ASC is a complex, turbulent fea- ture and similar to other major frontal currents, its effectiveness as a barrier to transport is variable in both space and time [see Fig. 1 and Bower et al., 1985]. In some areas of the Antarctic continental shelf, a shallow current flows to the west, poleward of the con- tinental shelf break. This feature, known as the Antarctic Coastal Current, often follows the edge of ice shelves, for example in the southern Weddell Sea [Nicholls et al., 2009], or –3– Confidential manuscript submitted to Reviews of Geophysics close to the coast, for example in the West Antarctic Peninsula (WAP) [Moffat and Mered- ith, 2018]. For succinctness, this review will focus only on the ASC. Around Antarctica, the strongest gradients in water mass properties typically occur between shelf waters and the relatively warm and salty water that comprises Circumpo- lar Deep Water (CDW) [Jacobs, 1991; Baines and Condie, 1998; Whitworth et al., 1998]; this gradient is the Antarctic Slope Front (ASF). In this article, we will at times refer to both the ASC and the ASF, however, in general, reference to the ASC implies the cou- pled circulation and frontal system. The properties of CDW are largely determined by processes occurring in ocean basins north of the Southern Ocean. The warm CDW then rises along density surfaces as it crosses the ACC and circulates through the sub-polar gyres of the Southern Ocean until it eventually sits at depths comparable to the sea floor of the continental shelf. The depth of the continental shelf is roughly 500 m around much of Antarctica, although deeper troughs play a key role in shelf-slope exchange. In addi- tion to gradients in temperature and salinity, the ASF may also support cross-slope gradi- ents in density. These gradients are physically realized as density surfaces that tilt away from horizontal. The geometry of these surfaces, or isopycnals, influences which den- sity classes have access to, or “ventilate,” the shelf, and which density classes intersect, or “incrop,” on the seafloor [Meredith et al., 2011; Dutrieux et al., 2014] (§2). A density class refers to waters within a range of densities, which typically have similar thermody- namic/chemical properties and are subject to similar physical processes. Isopycnal tilting is typically largest at the shelf break, where it also has the capacity for rapid change [Su et al., 2014]. Lateral density gradients are also associated with geostrophically-balanced, vertically-sheared along slope flows, such that the density field influences the vertical structure of the ASC’s along-slope flow (§3). Finally, lateral density gradients may be a source of potential energy that impacts the generation and intensity of ocean eddies, which in turn influence the transport of heat, freshwater, and other tracers [Moffat et al., 2009; Nøst et al., 2011; Stewart and Thompson, 2016].
Details
-
File Typepdf
-
Upload Time-
-
Content LanguagesEnglish
-
Upload UserAnonymous/Not logged-in
-
File Pages41 Page
-
File Size-