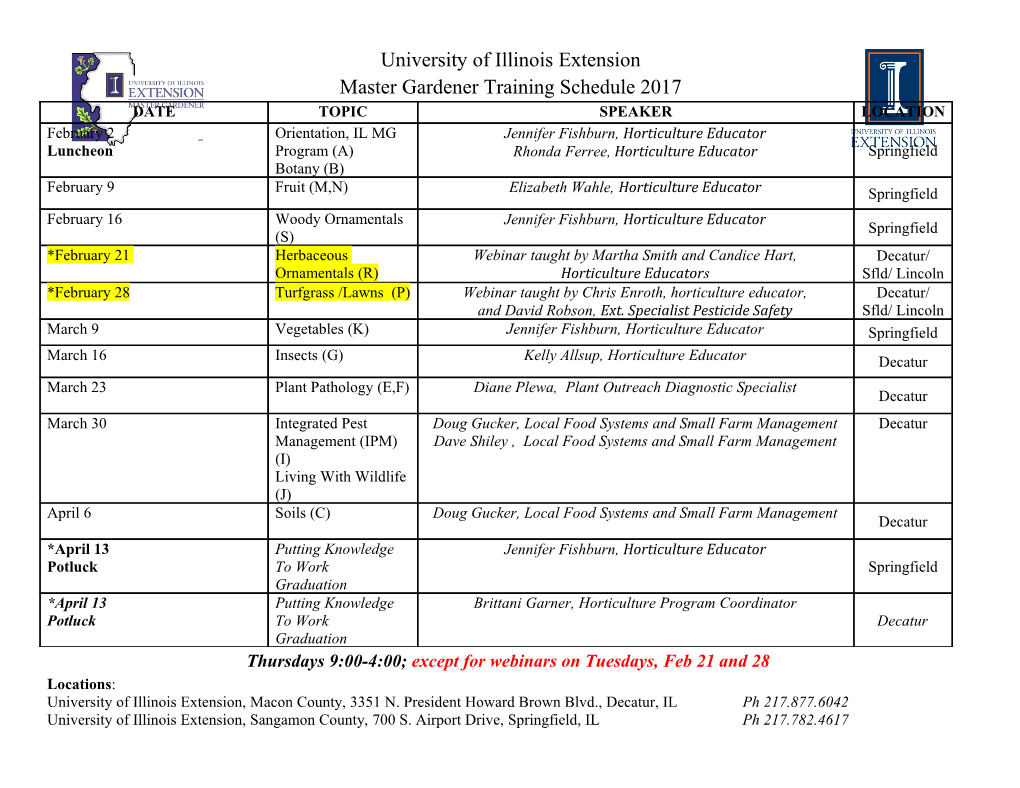
Cavity Ringdown Laser Spectroscopy (CRDS): History, Development, and Applications A. O’Keefe1, J. J. Scherer1, J.B. Paul2, and R.J. Saykally2 1 Los Gatos Research, 67 East Evelyn Ave., Mountain View, CA 94041 2 Department of Chemistry, University of California, Berkeley, CA 94720 Cavity Ringdown Spectroscopy has become a widely used technique in the optical absorption analysis of atoms, molecules, and optical components. The technique allows the determination of total optical losses within a closed cavity comprised of two or more mirrors, and can be made arbitrarily more sensitive by improvments in the cavity mirror reflectivity. Part of the great attraction that Cavity Ringdown has, in addition to its’ great sensitivity, is the simplicity of it’s use. The required equipment is modest, and the theory of operation is easily grasped by students of modest training. In fact, the technique is used at a growing number of universities as an undergraduate laboratory demonstration. This article presents a review of the development of the Cavity Ringdown technique from its’ roots as an unstable and difficult to use method of measuring mirror reflectivities, to the development of the high sensitivity pulsed and continuous adaptations which are in current use. Original Driving Forces: Ring Gyroscopes and Interferometers The fundamental concept behind the Cavity Ringdown technique can be traced back to several efforts in the early 1980’s which were aimed at characterizing the reflectivity of HR mirror surfaces for several different applications. The development of the optical ring gyroscope operating at the HeNe laser wavelength, 6328 Å, was having an enormous impact upon both civilian and military aviation systems. The accuracy of the navigational gyro was determined, in part, by the optical residence time within the ring cavity. Increasing the reflectivity of the mirror coatings was the obvious solution to this problem. The development of mirrors with reflectivities exceeding 99.9% was of great interest to the optics community as well as such coatings were of great value in interferometric applications. The problem confronting both communities was the lack of precision in the characterization of the mirror coatings which was unreliable below 0.1% change. This made it impossible to make the incremental improvments in coating quality. In 1980, Herbelin et al. (1) demonstrated a novel Phase Shift method which could accurately measure the averaged reflectivity of a pair of mirrors in a cavity to 0.01%. This was followed by the work of Anderson et al. (2) in 1984 which utilized a shuttered laser injection of an optical cavity and could determine the reflectivity of a mirror pair to an accuracy of 0.0005%. In this adaption of the measurment scheme, the output of a single mode Argon ion laser was passed through a pockels cell shutter, a polorizer, and a pair of mode matching lenses, into a closed, aligned ring cavity. A pair of mode matching lenses are used to optimize the injection coupling and to minimize the coupling and build up of multiple cavity spatial modes. The cavity can, in principle, be of any geometry as long as it is aligned. The use of a ring cavity rather than a two mirror linear cavity is advantageous in applications employing a continuous laser as this geometry largely eliminates optical feedback into the laser. Such feedback could result in laser instabilities. The laser cavity and the sample cavity are not locked to each other and so the laser cavity modes drift relative to the sample cavity modes. When the laser output frequency accidentally coincides with a sample cavity mode frequency, energy is efficiently coupled into the cavity. The build up of intra-cavity power is accompanied by an increased transmission out of the cavity in the “forward” direction, which is directed onto a photodetector. If left to drift independently, The photodetector would see a random sequence of transmission spikes of varying amplitude, reflecting the random temporal duration of the mode overlap. In Anderson’s adaption, a power level trigger senses when the intra-cavity power reaches a set level and signals the Pockels cell to shut off further laser injection. At this point the monitor detector records the transient response, or “ringdown” of the cavity. Assuming that the switching time of the Pockels cell is short relative to the cavity decay time, the measured output of the cavity decays exponentially according to the first order expression [-t/τ] I(t) = Io × exp (1) where τ is the ringdown decay time constant of the cavity. If the cavity loss is purely due to the transmission of the cavity mirrors, and assuming that the mirror transmission, T, is given by T ≈ 1 - R (2) then the cavity output decay time can be related to R via τ = L/c × [√R/(1-√R)] (3) where L is the mirror separation, c is the speed of light, and R = R1R2 is the reflectance for a two mirror configuration. While this approach demonstrated very good sensitivity, it suffered from temporal instability in that there was no precise method by which to control the onset of the measurement. Because the frequency coincidence of the laser and sample cavities was a random occurrance, it was impossible to synchronize to external experiments. Other factors contributed to the measured system sensitivity, however it was clear that with some effort the ultimate sensitivity could be improved. More important, from the standpoint of spectroscopic analysis, was the difficulty in frequency tuning a single mode laser over any usable or interesting range. While the approach of Anderson et al. (2) would be of value in testing mirror reflectivities at fixed frequencies, it was not yet ready for general spectroscopic use. Development of Pulsed Cavity Ringdown We adapted the approach of Anderson et al. (2) by substituting a tunable wavelength pulsed laser for the single mode fixed frequency laser. The first wavelength tunable cavity decay rate instruments were developed at Deacon Research for characterization of mirror reflectivities in an effort to help develop higher reflectivity mirrors for the early Free Electron Laser program. As mirrors of higher reflectivity were developed, it became clear that spectroscopic sensors based upon this technique would provide significant improvements over existing approaches, especially if mirror technology continued to improve. Deacon Research produced and sold dozens of mirror testing instruments based upon this technology under the commercial name Cavity Lossmeter. Most of these systems were sold to aerospace technology companies and several national laboratories for optic analysis, however some were adapted for spectroscopic use as the applications became apparent. The modifications made to the approach used by Anderson et al. (2) allowed us to overcome two problems. First, by using a pulsed laser with a short coherence length, light is coupled into the sample cavity with a constant efficiency (efficiency = 1- R, where R is the input mirror reflectivity). Under transient impulsive excitation, the cavity can accept the full spectral range of the input pulse and does not exhibit the narrow frequency acceptance of a classic etalon under continuous irradiation. The use of a pulsed dye laser source provided other advantages as well. The injection pulse could also be tuned in wavelength over the entire range of the dye laser. This is typically at least as wide as the bandwidth of the mirrors used in the cavity. This allowed not only the measurement of the mirror reflectivity at a single wavelength, but to map out the reflectivity curve of the coating. This information, along with the absolute value of the reflectivity, was important feedback to the coating manufacturers, and permitted a significant increase in coating reflectivity in only a few years. Using the Cavity Lossmeter to characterize and perfect new coatings helped produce a 100 fold increase in coating reflectivity in this period. This remarkable improvement in mirror coating reflectivity set the stage for the advent of Cavity Ringdown spectroscopy. During 1987 and 1988 a series of internal experiments was initiated at Deacon Research using a modified Lossmeter system to make total loss measurement of gas samples. Using a modest resolution pulsed dye laser (bandwidth ~ 1 cm-1) sytem we began a series of experiments aimed at demonstrating the sensitivity of the approach for a range of applications including static gas samples, gas phase reaction product analysis, and transient species detection. These tests utilized a large set of mirrors which spanned the spectral region from 295 nm to 1600 nm in regular intervals, with each mirror set having maximum reflectivities of ~ 99.99% or better. General Theory of Operation The theory of operation behind the pulsed Cavity Ringdown technique is quite simple (3-5). The basic theory is a special case of that developed by Anderson et al. (2) for the case of continuous wave excitation of a cavity, which is somewhat more complicated because in the continuous wave case the energy within the cavity builds up in time with the same time constant as the cavity decay (as long as the two cavity modes remain locked). When the source injection light is shut off rapidly relative to the cavity buildup or decay time, the resulting decay can be shown to be exponential. Within the constraints of pulsed excitation it is easy to demonstrate why this is so. The technique can be schematically illustrated as in Figure 1. The output of a tunable pulsed laser is spatially mode matched and injected into an optical cavity made of two or more mirrors with reflectivity R. The mirrors which make up the cavity must be dielectric multilayer reflectors as it is neccessary to allow for a small amout of transmission through the optic. Optical injection is accomplished by aligning the laser beam with the cavity axis and onto one of the cavity mirrors such that the laser beam is reflected back onto itself.
Details
-
File Typepdf
-
Upload Time-
-
Content LanguagesEnglish
-
Upload UserAnonymous/Not logged-in
-
File Pages21 Page
-
File Size-