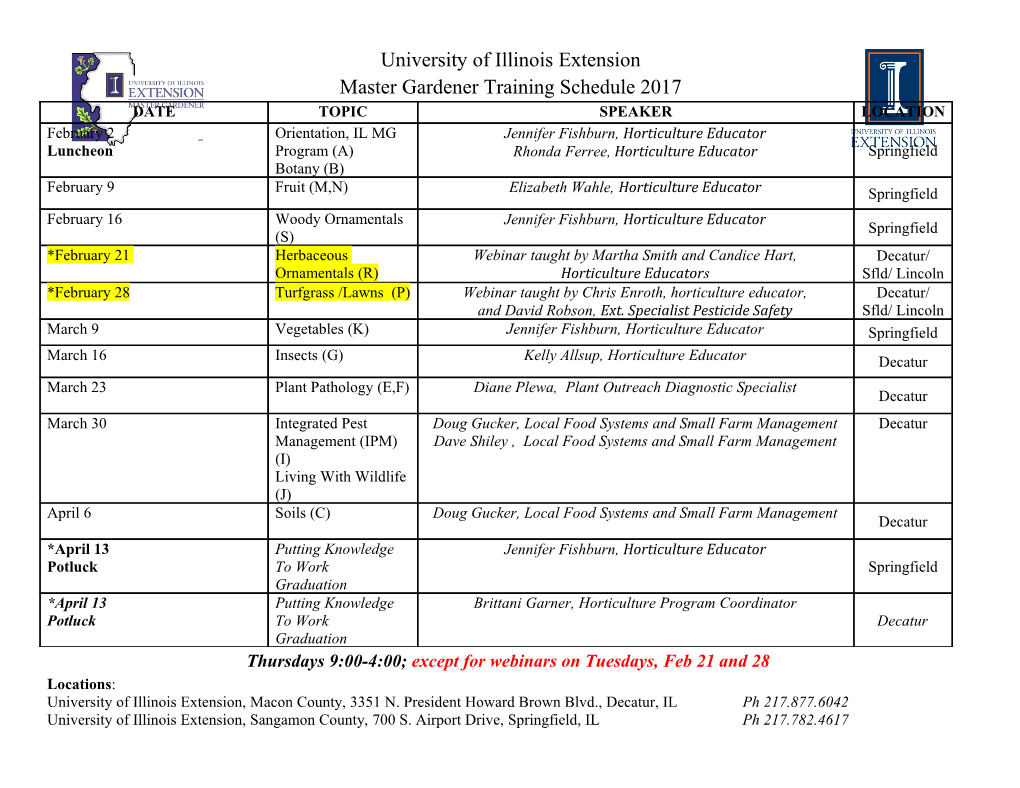
Amphiphile regulation of ion channel function by changes in the bilayer spring constant Jens A. Lundbæka,b,1, Roger E. Koeppe IIc, and Olaf S. Andersena aDepartment of Physiology and Biophysics, Weill Cornell Medical College, New York, NY 10065; bQuantum Protein Center, Department of Physics, Technical University of Denmark, Kongens Lyngby, DK-2800, Denmark; and cDepartment of Chemistry and Biochemistry, University of Arkansas, Fayetteville, AR 72701 Edited* by Christopher Miller, Brandeis University, Waltham, MA, and approved July 16, 2010 (received for review June 1, 2010) Many drugs are amphiphiles that, in addition to binding to a A B particular target protein, adsorb to cell membrane lipid bilayers and alter intrinsic bilayer physical properties (e.g., bilayer thick- ness, monolayer curvature, and elastic moduli). Such changes can modulate membrane protein function by altering the energetic F ΔG dis cost ( bilayer) of bilayer deformations associated with protein conformational changes that involve the protein-bilayer interface. d l d l But amphiphiles have complex effects on the physical properties of 0 0 ΔG lipid bilayers, meaning that the net change in bilayer cannot be F predicted from measurements of isolated changes in such proper- dis ties. Thus, the bilayer contribution to the promiscuous regulation Fig. 1. Hydrophobic coupling between a bilayer-embedded protein and its of membrane proteins by drugs and other amphiphiles remains host lipid bilayer. (A) A protein conformational change causes a local bilayer unknown. To overcome this problem, we use gramicidin A (gA) deformation. (B) Formation of a gA channel involves local bilayer thinning. channels as molecular force probes to measure the net effect of Modified from ref. 4. amphiphiles, at concentrations often used in biological research, on the bilayer elastic response to a change in the hydrophobic BIOPHYSICS AND length of an embedded protein. The effects of structurally diverse channels as probes to sense net changes in bilayer properties COMPUTATIONAL BIOLOGY amphiphiles can be described by changes in a phenomenological as experienced by a bilayer-spanning protein. H gA channels are dimers (D) formed by the transbilayer asso- bilayer spring constant ( B) that summarizes the bilayer elastic properties, as sensed by a bilayer-spanning protein. Amphiphile- ciation of monomeric subunits (M) from each bilayer leaflet H (Fig. 1B), and channel gating is described by induced changes in B, measured using gA channels of a particular length, quantitatively predict changes in lifetime for channels of a k — 1 different length as well as changes in the inactivation of voltage- 2M ⇄ D; dependent sodium channels in living cells. The use of gA channels k−1 as molecular force probes provides a tool for quantitative, predic- tive studies of bilayer-mediated regulation of membrane protein where k1 and k−1 are the association and dissociation rate con- function by amphiphiles. stants for the monomer ↔ dimer equilibrium. Channel formation in a bilayer with a hydrophobic thickness (d0) that exceeds the bilayer elasticity ∣ hydrophobic coupling ∣ hydrophobic matching channel hydrophobic length (l) involves a local bilayer deforma- tion (16–19) with an associated deformation energy. The bilayer, F t long has been suspected that “membrane-active” or “mem- in response, exerts a disjoining force ( dis) on the channel, the brane-stabilizing” drugs could regulate membrane protein func- magnitude of which is determined by the bilayer elastic properties I B F k tion by partitioning into the host lipid bilayer and thereby alter its (Fig. 1 ). Amphiphiles that decrease dis will increase 1, which is physical properties (1, 2). Indeed, numerous studies have shown reported as an increase in channel appearance frequency (f), and that amphiphiles, including many drugs, alter lipid bilayer physi- decrease k−1, which is reported as an increase in channel lifetime cal properties (for a recent review, see ref. 3). Moreover, mem- (τ ¼ 1∕k−1) (4, 12). brane protein function involves conformational changes at the Results and Discussion protein-bilayer boundary (4), which due to hydrophobic coupling A The amphiphile Triton X-100 (TX100) increases τ, meaning that will perturb the surrounding bilayer (Fig. 1 ). The associated F ΔG it decreases dis (12). Toquantify the changes in the bilayer elastic bilayer deformation energy ( bilayer) contributes to the free en- τ ergy of a protein conformational change (ΔG ), meaning that properties, we compare the changes in for gA channels formed prot by subunits of different length and chirality; the 13-residue changes in bilayer physical properties can alter protein function 1 2 − 1 by altering ΔG , cf. refs. 4–8. The promiscuous regulation of ½des-Val -Gly gA and the 15-residue ½Ala gA (20) [designated bilayer gA−ð13Þ and AgA(15), respectively]. Fig. 2A shows current traces membrane proteins by amphiphiles therefore may be due to μ − 13 amphiphile-induced changes in ΔG . This mechanism would that illustrate the effects of 3 M TX100 on gA ð Þ and AgA bilayer (15) channels in diphytanoylphosphatidylcholine ðDPhPCÞ∕n- provide a rationale for the observed correlations between the f τ increased hydrophobicity (or lipophilicity) of drugs and the like- decane bilayers. TX100 increases and for both channel types, lihood of adverse events (9) or attrition during drug development with the larger effects on the shorter channels. (10, 11). Amphiphilic molecules alter many different bilayer properties Author contributions: J.A.L. and O.S.A. designed research; J.A.L. and O.S.A. performed [including intrinsic curvature (12), thickness (13), and elastic research; R.E.K. contributed new reagents/analytic tools; J.A.L. and O.S.A. analyzed data; moduli (14, 15)], some of which may have opposing effects on and J.A.L., R.E.K., and O.S.A. wrote the paper. the bilayer deformation energy (4). This complicates attempts The authors declare no conflict of interest. ΔG to predict even the sign of the changes in bilayer (12). The pro- *This Direct Submission article had a prearranged editor. blem can be overcome, however, by using gramicidin A (gA) 1To whom correspondence should be addressed. E-mail: [email protected]. www.pnas.org/cgi/doi/10.1073/pnas.1007455107 PNAS Early Edition ∣ 1of4 Downloaded by guest on September 29, 2021 ∆ ∆ Fig. 2B shows the concentration-dependent effects of TX100 A ∆G ∆GkTB G GkT and six other amphiphiles [capsaicin, capsazepine (12), daidzein, 0123 01234 genistein, phloretin (21), and GsMTx4 (22)], on τ for gA channels formed by (left- or right-hand) subunits of 13 residues (τ13)or15 3 3 ∆ G residues (τ15). At concentrations where these amphiphiles alter 2 2 ∆ membrane protein function (summarized in the original articles), G they all increase τ for both channel types—and all with the larger effects on the shorter channels; cf. refs. 4, 12, 21, 22. [At the con- τ/τ 1 1 k centrations used in the gA channel experiments, the amphiphiles T do not alter membrane capacitance (12, 21, 23). Thus changes in 0 0 bilayer thickness cannot account for the effects.] 01234 The changes in channel lifetimes reflect changes in activation C τ/τ energy for channel dissociation (ΔG‡): 3 3 ∆ G D ΔG‡ − ΔG‡ τ∕τ k T; [1] 10 cntl ¼ lnf cntlg · B ∆ 2 2 G where k and T are, respectively, Boltzmann’s constant and the 5 B τ/τ 1 1 temperature in Kelvin, and “cntl” denote results in the absence of kT amphiphile. The larger changes in lifetime for the shorter chan- 0 ΔG‡ 0 0 012 nels relative to the longer channels mean that the changes in ∆∆ ∆∆ GG13/ 15 also are larger for the shorter channels than for the longer chan- 0123 nels (Fig. 3). Moreover, because the changes in τ13 and τ15 result τ/τ ‡ from the same underlying mechanism, the changes τ (in ΔG13 and ΔG‡ A Fig. 3. Effects of amphiphiles on lifetimes of channels formed by 13-residue 15) are likely to be correlated (5). Fig. 3 shows the changes in subunits (expressed as lnfτ∕τ g, left axis, or activation energy, ΔG‡ − ΔG‡ , ‡ ‡ cntl cntl ΔG13 as a function of the changes in ΔG15 in experiments with right axis) vs. corresponding effects on channels formed by 15-residue sub- DPhPC as the bilayer-forming lipid. Despite the very different units (bottom or top axis). (A) Effects of TX100, capsaicin, capsazepine, daid- structures of the amphiphiles, the changes are described by a zein, genistein, phloretin, or GsMTx4 in DPhPC∕n-decane bilayers (except for ΔΔG‡ ∕ΔΔG‡ 1 19 TX100, results from refs. 12, 21 and 22). A subset of the results was published shared linear relation with a slope: 13 15 ¼ . Æ previously (5). (B) Effects of TX100, curcumin, docosahexaenoic acid (DHA), 2 0.02 (ÆSE, r > 0.99). A similar relation is obtained using a 2,3-butanedione monoxime (BDM), reduced Triton X-100 (rTX100), Z-Gly- number of other amphiphiles with dioleoylphosphatidylcholine D-Phe (ZGdF), and Z-Gly-Phe (ZGF) (at pH 7 or pH 4) and Z-D-Phe-Phe-Gly (DOPC) as the bilayer-forming lipid. Fig. 3B shows the effects (ZdFFG, at pH 7) in DOPC∕n-decane bilayers (except for TX100, results from refs. 24–28). (C) Superimposition of results obtained using DPhPC (red) or of TX100 and seven other amphiphiles [curcumin (24), docosa- ‡ ‡ DOPC (black). (D) Distribution of ΔΔG13∕ΔΔG15 for individual amphiphiles using DPhPC (red) or DOPC (black). Mean Æ SEM (n ≥ 3)orÆ range (n ¼ 2). hexaenoic acid (25), 2,3-butanedione monoxime (26), reduced Triton X-100 (27); and the viral antifusion peptides Z-Gly-D- Phe, Z-Gly-Phe and Z-D-Phe-Phe-Gly (28)]. Again the results ‡ ‡ can be described by a shared linear relation: ΔΔG13∕ΔΔG15 ¼ 1.21 Æ 0.05 (ÆSE, r2 ¼ 0.95). Indeed, the DPhPC and DOPC re- sults can be superimposed (Fig.
Details
-
File Typepdf
-
Upload Time-
-
Content LanguagesEnglish
-
Upload UserAnonymous/Not logged-in
-
File Pages4 Page
-
File Size-