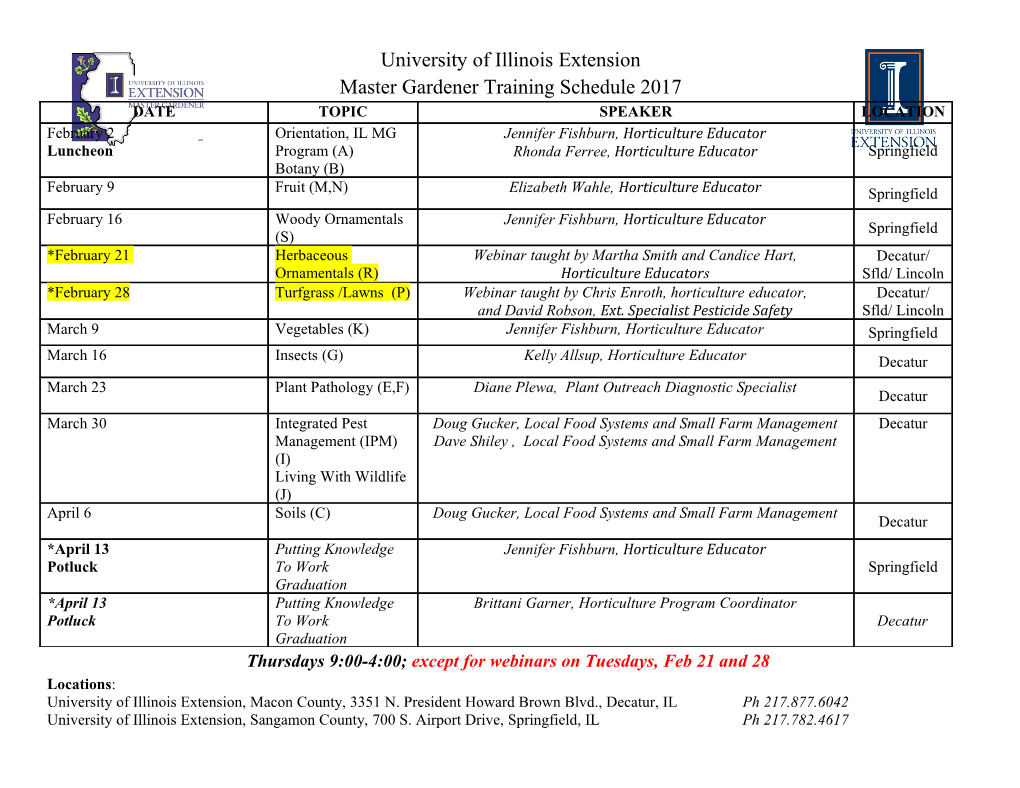
“conti” — 2004/9/6 — 9:53 — page 1 — #1 CONTINUUM MECHANICS lecture notes 2003 jp dr.-ing. habil. ellen kuhl technical university of kaiserslautern “conti” — 2004/9/6 — 9:53 — page 2 — #2 “conti” — 2004/9/6 — 9:53 — page i — #3 Contents 1 Tensor calculus 1 1.1 Tensor algebra . 1 1.1.1 Vector algebra . 1 1.1.1.1 Notation . 1 1.1.1.2 Euclidian vector space . 2 1.1.1.3 Scalar product . 4 1.1.1.4 Vector product . 5 1.1.1.5 Scalar triple vector product . 6 1.1.2 Tensor algebra . 7 1.1.2.1 Notation . 7 1.1.2.2 Scalar products . 10 1.1.2.3 Dyadic product . 12 1.1.2.4 Scalar triple vector product . 13 1.1.2.5 Invariants of second order tensors . 14 1.1.2.6 Trace of second order tensors . 15 1.1.2.7 Determinant of second order tensors . 16 1.1.2.8 Inverse of second order tensors . 17 1.1.3 Spectral decomposition . 18 1.1.4 Symmetric – skew-symmetric decomposition . 19 1.1.4.1 Symmetric tensors . 20 1.1.4.2 Skew-symmetric tensors . 21 1.1.5 Volumetric – deviatoric decomposition . 22 1.1.6 Orthogonal tensors . 23 1.2 Tensor analysis . 24 1.2.1 Derivatives . 24 1.2.1.1 Frechet derivative . 24 1.2.1.2 Gateaux derivative . 24 1.2.2 Gradient . 25 1.2.2.1 Gradient of a scalar–valued function . 25 1.2.2.2 Gradient of a vector–valued function . 25 1.2.3 Divergence . 26 1.2.3.1 Divergence of a vector field . 26 1.2.3.2 Divergence of a tensor field . 26 1.2.4 Laplace operator . 27 1.2.4.1 Laplace operator acting on scalar–valued function . 27 1.2.4.2 Laplace operator acting on vector–valued function . 27 i “conti” — 2004/9/6 — 9:53 — page ii — #4 Contents 1.2.5 Integral transformations . 29 1.2.5.1 Integral theorem for scalar–valued fields . 29 1.2.5.2 Integral theorem for vector–valued fields . 29 1.2.5.3 Integral theorem for tensor–valued fields . 29 2 Kinematics 30 2.1 Motion . 30 2.2 Rates of kinematic quantities . 31 2.2.1 Velocity . 31 2.2.2 Acceleration . 31 2.3 Gradients of kinematic quantities . 32 2.3.1 Displacement gradient . 32 2.3.2 Strain . 34 2.3.3 Rotation . 35 2.3.4 Volumetric–deviatoric decomposition of strain tensor . 37 2.3.5 Strain vector . 41 2.3.6 Normal–shear decomposition . 42 2.3.7 Principal strains – stretches . 43 2.3.8 Compatibility . 44 2.3.9 Special case of plane strain . 45 2.3.10 Voigt representation of strain . 45 3 Balance equations 46 3.1 Basic ideas . 46 3.1.1 Concept of mass flux . 48 3.1.2 Concept of stress . 50 3.1.2.1 Volumetric–deviatoric decomposition of stress tensor . 54 3.1.2.2 Normal–shear decomposition . 57 3.1.2.3 Principal stresses . 58 3.1.2.4 Special case of plane stress . 59 3.1.2.5 Voigt representation of stress . 59 3.1.3 Concept of heat flux . 60 3.2 Balance of mass . 62 3.2.1 Global form of balance of mass . 62 3.2.2 Local form of balance of mass . 63 3.2.3 Classical continuum mechanics of closed systems . 63 3.3 Balance of linear momentum . 64 3.3.1 Global form of balance of momentum . 64 3.3.2 Local form of balance of momentum . 64 3.3.3 Reduction with lower order balance equations . 65 3.3.4 Classical continuum mechanics of closed systems . 66 3.4 Balance of angular momentum . 66 3.4.1 Global form of balance of angular momentum . 66 3.4.2 Local form of balance of angular momentum . 67 3.4.3 Reduction with lower order balance equations . 68 ii “conti” — 2004/9/6 — 9:53 — page iii — #5 Contents 3.5 Balance of energy . 69 3.5.1 Global form of balance of energy . 69 3.5.2 Local form of balance of energy . 70 3.5.3 Reduction with lower order balance equations . 70 3.5.4 First law of thermodynamics . 71 3.6 Balance of entropy . 73 3.6.1 Global form of balance of entropy . 73 3.6.2 Local form of balance of entropy . 74 3.6.3 Reduction with lower order balance equations . 74 3.6.4 Second law of thermodynamics . 75 3.7 Generic balance equation . 77 3.7.1 Global / integral format . 77 3.7.2 Local / differential format . 77 3.8 Thermodynamic potentials . 79 4 Constitutive equations 80 4.1 Linear constitutive equations . 81 4.1.1 Mass flux – Fick’s law . 82 4.1.2 Momentum flux – Hook’s law . 83 4.1.3 Heat flux – Fourier’s law . 85 4.2 Hyperelasticity . 86 4.2.1 Specific stored energy . 86 4.2.2 Specific complementary energy . 88 4.3 Isotropic hyperelasticity . 89 4.3.1 Specific stored energy . 89 4.3.2 Specific complementary energy . 95 4.3.3 Elastic constants . 99 4.4 Transversely isotropic hyperelasticity . 100 A Ub¨ ungsaufgaben 105 A.1 Tensoralgebra zweistufiger Tensoren . 105 A.2 Tensoranalysis . 112 A.3 Ableitungen . 114 A.4 Verzerrungs– und Spannungsvektor . 117 iii “conti” — 2004/9/6 — 9:53 — page iv — #6 “conti” — 2004/9/6 — 9:53 — page 1 — #7 1 Tensor calculus 1.1 Tensor algebra 1.1.1 Vector algebra 1.1.1.1 Notation Einstein’s summation convention 3 ui ∑j 1 Ai j x j bi Ai j x j bi (1.1.1) summation over indices that appear twice in a term or sym- bol, with silent (dummy) index j and free index i, and thus u1 A11 x1 A12 x2 A13 x3 b1 u2 A21 x1 A22 x2 A23 x3 b2 (1.1.2) u3 A31 x1 A32 x2 A33 x3 b3 Kronecker symbol δi j 1 for i j δi j (1.1.3) 0 for i j multiplication with Kronecker symbol corresponds to ex- change of silent index with free index of Kronecker symbol ui δi j u j (1.1.4) 1 “conti” — 2004/9/6 — 9:53 — page 2 — #8 1 Tensor calculus 3 permutation symbol ei jk 1 for i, j, k ... even permutation 3 ei jk 1 for i, j, k ... odd permutation (1.1.5) 0 for ... else 1.1.1.2 Euclidian vector space consider linear vector space 3 characterized through ad- dition of its elements u, v and multiplication with real scalars α, β α, β ... real numbers u, v 3 3 ... linear vector space definition of linear vector space 3 through the following axioms α u v α u α v α β u α u β u (1.1.6) α β u α β u zero element and identity 0 u 0 1 u u (1.1.7) 3 linear independence of elements e1, e2, e3 if α1 α2 α3 0 is the only (trivial) solution to αi ei 0 (1.1.8) 2 “conti” — 2004/9/6 — 9:53 — page 3 — #9 1.1 Tensor algebra consider linear vector space 3 equipped with a norm n u mapping elements of the linear vector space 3 to the space of real numbers n : 3 norm (1.1.9) definition of norm through the following axioms n u 0 n u 0 u 0 n α u α n u (1.1.10) n u v n u n v n2 u v n2 u v 2 n2 u n2 v consider Euclidian vector space 3 equipped with the Eu- clidian norm 2 2 2 1 2 n u u u u u1 u2 u3 (1.1.11) mapping elements of the Euclidian vector space 3 to the space of real numbers n : 3 Euclidian norm (1.1.12) representation of three–dimensional vector a 3 a ai ei a1 e1 a2 e2 a3 e3 (1.1.13) with a1, a2, a3 coordinates (components) of a relative to the basis e1, e2, e3 t a a1, a2, a3 (1.1.14) 3 “conti” — 2004/9/6 — 9:53 — page 4 — #10 1 Tensor calculus 1.1.1.3 Scalar product Euclidian norm enables the definition of scalar (inner) prod- uct between two vectors u, v and introduces a scalar α u v α (1.1.15) geometric interpretation with 0 ϑ π being the angle u enclosed by the vectors u and v, ϑ then u cos ϑ can be inter- preted as the projection of u u cos ϑ v onto the direction of v andPSfrag replacements u cos ϑ u v u v cos ϑ corresponds to the grey area in the picture with the above interpretation with 0 ϑ π, obviously u v u v (1.1.16) properties of scalar product u v v u α u β v w α u w β v w (1.1.17) w α u β v α w u β w v positive definiteness of scalar product u u 0, u u 0 u 0 (1.1.18) orthogonal vectors u and v u v 0 u v (1.1.19) 4 “conti” — 2004/9/6 — 9:53 — page 5 — #11 1.1 Tensor algebra 1.1.1.4 Vector product vector.
Details
-
File Typepdf
-
Upload Time-
-
Content LanguagesEnglish
-
Upload UserAnonymous/Not logged-in
-
File Pages112 Page
-
File Size-