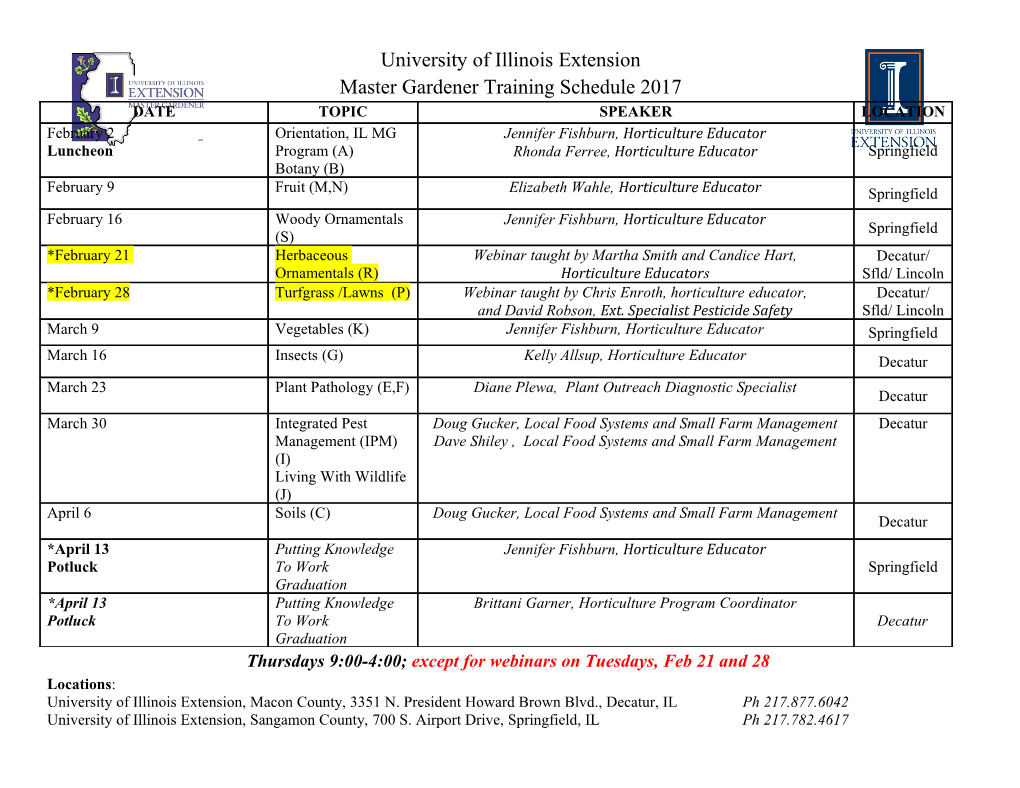
Sheaf Cohomology Gabriel Chˆenevert Payman Kassaei September 24, 2003 In this lecture, we define the cohomology groups of a topological space X with coefficients in a sheaf of abelian groups F on X in terms of the derived functors of the global section functor Γ(X; ¢ ). Then we introduce Cechˇ coho- mology with respect to an open covering of X, which permits to make explicit calculations, and discuss under which conditions it can be used to compute sheaf cohomology. 1 Derived functors We first need to review some homological algebra in order to be able to define sheaf cohomology using the derived functors of the global sections functor. Let A be an abelian category, that is, roughly, an additive category in which there exist well-behaved kernels and cokernels for each morphism, so that, for example, the notion of an exact sequence in A makes sense. If X is a fixed object in A and Ab denotes the category of abelian groups, then we have a contravariant functor Hom( ¢ ;X): A ¡! Ab: It is readily seen to be left exact, that is, for any short exact sequence f g 0 ¡! A ¡! B ¡! C ¡! 0 in A , the sequence g¤ f ¤ 0 ¡! Hom(C; X) ¡! Hom(B; X) ¡! Hom(A; X) is exact in Ab. Definition 1.1. An object I of A is said to be injective if the functor Hom( ¢ ;I) is exact. 1 Since Hom( ¢ ;I) is always left exact, we see that an object I of A is injective if and only if for each exact sequence 0 ! A ! B and morphism A ! I, there exists a morphism B ! I making the following diagram commute. 0 / A / B ~ ~ ~ ² Ä~ I That is, morphisms to an injective defined on a sub-object A can always be extended to the whole object B. Definition 1.2. An abelian category A is said to have enough injectives if each object of A can be embedded in an injective object. This is equivalent to saying that each object A of A admits an injective resolution, that is, a long exact sequence 0 ¡! A ¡! I0 ¡! I1 ¡! I2 ¡! ¢ ¢ ¢ where each Ii is injective. To see this, first embed A in an injective I0, then embed the cokernel of the inclusion " : A ! I0 in an injective I1, and take for I0 ! I1 the composite I0 ! Coker " ! I1, and so on. Definition 1.3. A(cochain) complex A² in an abelian category A is a collection of objects Ai of A , i 2 Z, together with morphisms di : Ai ! Ai+1 such that di+1 ± di = 0 for all i. The maps di are called the differentials or coboundary maps of the complex A. To any complex A² in A one can associate a complex H²(A²) with zero differential, called the cohomology of A², by defining for each i 2 Z Hi(A²) := Ker di=Im di¡1 (the condition that di ± di¡1 = 0 ensures that this definition makes sense). A morphism of complexes f : A² ! B² is a collection of maps f i : Ai ! Bi which commute with the differentials, i.e. that make the following diagram commutative. di¡1 di ¢ ¢ ¢ / Ai¡1 / Ai / Ai+1 / ¢ ¢ ¢ f i¡1 f i f i+1 ² ² ² ¢ ¢ ¢ / Bi¡1 / Bi / Bi+1 / ¢ ¢ ¢ di¡1 di 2 Any such morphism induces a morphism H²(f): H²(A²) ! H²(B²) on the cohomology, defined on Hi(A²) by Hi(f)(a + Im di) := f i(a) + Im di: Thus, we may think of H² as a functor on the category of complexes in A . One of the main properties of this functor is the following. Proposition 1.4. Let A be an abelian category and 0 ¡! A² ¡! B² ¡! C² ¡! 0 a short exact sequence of complexes in A . Then we get a natural long exact sequence 0 ! H0(A²) ! H0(B²) ! H0(C²) ! H1(A²) ! H1(B²) ! H1(C²) ! ::: in cohomology. Proof. Use the “snake lemma” (e.g. [W, Th. 1.3.1]). Definition 1.5. Two morphisms of complexes f; g : A² ! B² are homotopic if there exists a k : A² ! B² of degree ¡1 such that f ¡ g = dk + kd. Note that k is simply a collection of morphisms from Ai ! Bi¡1 for each integer i. It is direct to see that if f and g are homotopic, they induce the same morphism on the cohomology, i.e. H²(f) = H²(g). Now, let’s write an injective resolution 0 ¡! A ¡! I0 ¡! I1 ¡! I2 ¡! ¢ ¢ ¢ as 0 ! A ! I², where we think of I² as a complex which is 0 in negative degrees. Let A be an abelian category with enough injectives and F : A ! B an (additive) covariant left exact functor. Choose an injective resolution 0 ! A ! I² of each object A of A . Then forget A itself to retain only the complex I². If we apply F we still get a complex F (I²), so that we can define RiF (A) := Hi(F (I²)): The key point which makes this definition work is the following lemma, which relies on the property of being injective. 3 Lemma 1.6. Let 0 ! B ! I² be an injective resolution of an object B and 0 ! A ! J ² any resolution of A. Then any morphism f : A ! B induces a morphism of complexes f ² : J ² ! I², which is unique up to homotopy. This implies, first of all, that any two injective resolutions 0 ! A ! I² and 0 ! B ! J ² of the same object A are homotopy equivalent, thus it is also the case for the complexes F (I²) and F (J ²), which guarantees that Hi(F (I²)) =» Hi(F (J ²)) for all i. This means that RiF (A) is well defined (up to canonical isomorphism). Second, any morphism f : A ! B in A induces a morphism of complexes f ² : I² ! J ² between injective resolutions, so that we get a morphism RiF (f) := Hi(F (f ²)) : RiF (A) ¡! RiF (B) at the level of cohomology. Proposition 1.7. If A has enough injectives and F : A ! B is a left exact functor, then for each i ¸ 0 RiF : A ¡! B as defined above is an additive functor, called the ith right derived functor of F. Moreover, R0F =» F , and each short exact sequence 0 ¡! A ¡! B ¡! C ¡! 0 in A induces a natural long exact sequence 0 ! F (A) ! F (B) ! F (C) ! R1F (A) ! R1F (B) ! R1F (C) ! ¢ ¢ ¢ in B. Proof. The fact that each RiF is an additive functor follows from Lemma 1.6. To verify that R0F =» F , choose an injective resolution 0 0 ¡! A ¡!" I0 ¡!d I1 ¡! ¢ ¢ ¢ of A. Since F is left exact, we know that F (") F (d0) 0 ¡! F (A) ¡! F (I0) ¡! F (I1) is still exact. Thus we find that R0F (A) = Ker F (d0)=Im 0 = Ker F (d0) = Im F (") =» F (A): 4 Similarly, if f is a morphism in A , it is easy to verify that R0F (f) corresponds to F (f) via this isomorphism. Now, let 0 ¡! A ¡! B ¡! C ¡! 0 be any short exact sequence in A . Choose injective resolutions 0 ! A ! I² and 0 ! C ! K². Then, the “horseshoe lemma” (see e.g. [W, Th. 2.2.8]) implies that there exists an injective resolution 0 ! B ! J ² of B so that our short exact sequence induces a short exact sequence of complexes 0 ¡! I² ¡! J ² ¡! K² ¡! 0: Applying F , this sequence remains exact (because the Ii’s are injective), and the short exact sequence of complexes 0 ¡! F (I²) ¡! F (J ²) ¡! F (K²) ¡! 0 induces in cohomology the appropriate long exact sequence using Proposition 1.4 and the fact that R0F =» F just proved. Proposition 1.8. If F : A ! B is any left exact functor and I is injective, then RiF (I) = 0 for all i > 0. Proof. Use the injective resolution 0 ! I ! I ! 0 to compute RiF (I). Remark 1.9. The collection of functors fRiF g forms what is called a ±-functor. Let A and B be abelian categories. A (covariant) ±-functor from A to B is a collection of (covariant) functors fF ig for each i ¸ 0 such that a short exact sequences of objects (functorially) gives rise to a long exact sequence as in Proposition 1.7. (The name ±-functor relates to the connecting homomorphisms in the long exact sequence, which are often denoted by ±). A ±-functor fF ig is universal if for any other ±-functor fGig, any morphism F 0 ! G0 can be uniquely completed to morphisms F i ! Gi, commuting with ±. Therefore, there is at most one universal ±-functor which in degree 0 is a given functor. If the covariant ±-functor fF ig has the property that for any object A of A there is some monomorphism u : A ! M such that F i(u) = 0 for all i > 0, then fF ig is universal. This implies that the right derived functors of a left exact covariant functor form a universal ±-functor (since one can take u to be the injection of A in an injective object, as A has enough injectives). In particular, if A has enough injectives, and fGig : A!B is a universal ±-functor such that G0 is left exact, then we have Gi = RiG0. 5 Remark 1.10. If F is exact, then RiF (A) = 0 for any A and i > 0. Indeed, if we choose an injective resolution 0 ! A ! I², the complex F (I²) is exact in degrees i > 0 since F is exact, thus RiF (A) = Hi(F (I²)) = 0 for i > 0. Definition 1.11. An object A of A is F -acyclic if RiF (A) = 0 for all i > 0.
Details
-
File Typepdf
-
Upload Time-
-
Content LanguagesEnglish
-
Upload UserAnonymous/Not logged-in
-
File Pages20 Page
-
File Size-