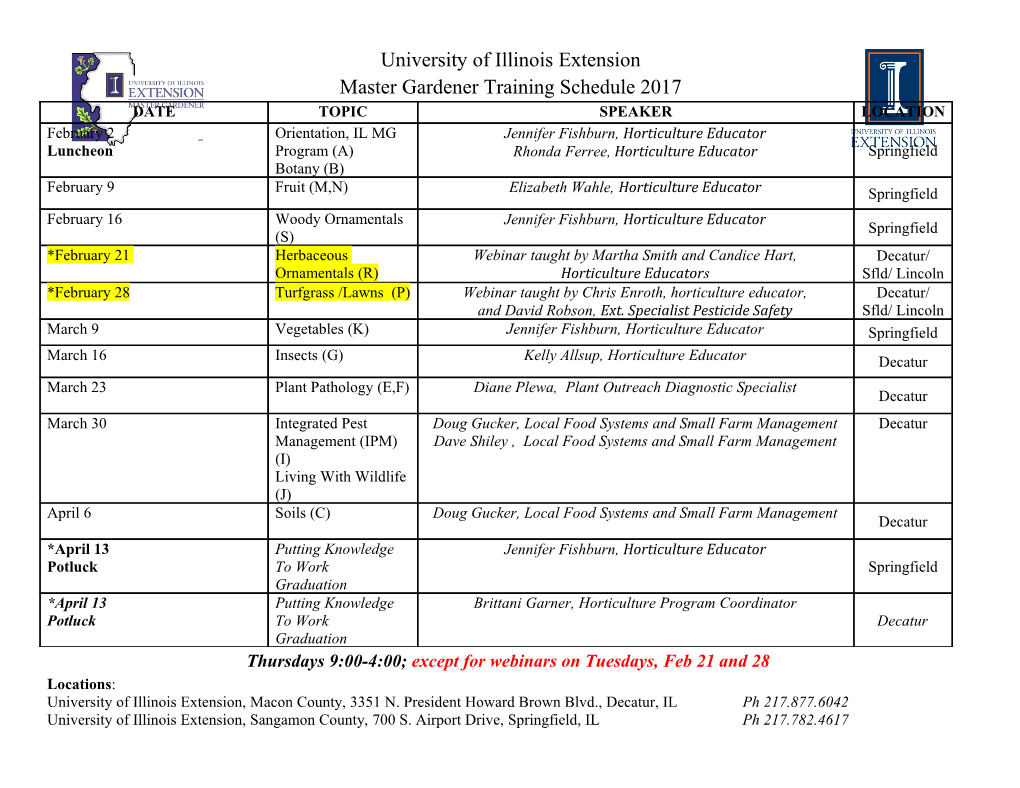
Dynamic controls on erosion and deposition on debris-fl ow fans Peter Schürch1,2*, Alexander L. Densmore1, Nicholas J. Rosser1, and Brian W. McArdell2 1Department of Geography and Institute of Hazard, Risk, and Resilience, Durham University, South Road, Durham DH1 3LE, UK 2Federal Institute for Forest, Snow and Landscape Research (WSL), Zürcherstrasse 111, 8903 Birmensdorf, Switzerland ABSTRACT channel bed by systematically measuring ero- Debris fl ows are among the most hazardous and unpredictable of surface processes in sion and deposition in a series of natural fl ows at mountainous areas. This is partly because debris-fl ow erosion and deposition are poorly both the reach and fan scales. We hypothesize, understood, resulting in major uncertainties in fl ow behavior, channel stability, and sequen- based on the results of Berger et al. (2011), that tial effects of multiple fl ows. Here we apply terrestrial laser scanning and fl ow hydrograph local bed elevation change is related to basal analysis to quantify erosion and deposition in a series of debris fl ows at Illgraben, Switzer- shear stress (and thus to maximum fl ow depth) land. We identify fl ow depth as an important control on the pattern and magnitude of ero- and fl ow volume. We use a terrestrial laser scan- sion, whereas deposition is governed more by the geometry of fl ow margins. The relationship ner (TLS) to determine high-resolution reach- between fl ow depth and erosion is visible both at the reach scale and at the scale of the entire scale measurements of erosion and deposition fan. Maximum fl ow depth is a function of debris-fl ow front discharge and pre-fl ow channel in a natural channel caused by four debris fl ows. cross-section geometry, and this dual control gives rise to complex interactions with implica- We then relate these data both to fl ow depth and tions for long-term channel stability, the use of fan stratigraphy for reconstruction of past to fan-scale fl ow volume changes estimated debris-fl ow regimes, and the predictability of debris-fl ow hazards. from debris-fl ow hydrographs. INTRODUCTION stream channel widening (Cannon, 1989; Fan- STUDY AREA Debris fl ows are ubiquitous hazards in moun- nin and Wise, 2001), or fl ow volume, based on The Illgraben debris-fl ow fan is in the Rhone tain areas, not least because of their ability to an observed power-law relationship between valley, Switzerland (Fig. 1), and has a long his- avulse from an existing channel and inundate fl ow volume and total inundated area (Griswold tory of debris fl ows (Marchand, 1871; Lichten- adjacent areas on debris-fl ow fans (Rickenmann and Iverson, 2007). More generally, detailed hahn, 1971). The fan has an area of 6.6 km2 with and Chen, 2003; Jakob and Hungr, 2005). The monitoring of experimental fl ows (Major and a radius of 2 km and a gradient that decreases avulsion probability is controlled mainly by the Iverson, 1999) and physically based descrip- from 10% to 8% downfan (Schlunegger et al., ratio of fl ow peak discharge and channel convey- tion of fl uid-solid mixtures (Iverson, 1997) have 2009). The bedrock geology in the catchment ance capacity. While the latter can be estimated related fl ow mobility to granular temperature, is dominated by schist, dolomite breccia, and from fi eld measurements (Whipple and Dunne, defi ned as the mean square of particle veloc- quartzite (Gabus et al., 2008). The lowermost 1992), both parameters can change rapidly dur- ity fl uctuations, and excess pore-fl uid pressure bedrock along the Illgraben channel crops out ing a fl ow due to erosion and deposition along (McCoy et al., 2010). These effects are counter- just below a sediment retention dam (check the fl ow path (Fannin and Wise, 2001). This not acted by friction at the dry coarse-grained fl ow dam, CD1, Fig. 1); downstream the channel bed only makes it diffi cult to predict the temporal margins (Major and Iverson, 1999). consists of unconsolidated sediments. Convec- evolution of an individual fl ow, but also changes The objective of this study is to understand tive storms from May to September trigger three the boundary conditions for the next fl ow in that the interaction between a debris fl ow and the to fi ve debris fl ows per year (McArdell et al., channel. There results a critical need to under- stand the dynamic relationships and feedbacks between debris-fl ow volume and the changes in 7°37′E7°38′E7°39′E7°40′E channel topography due to erosion and deposi- tion as the fl ows traverse a fan. ′ 46°19 N 29 Previous studies have focused more on 650 m Rhone debris-fl ow deposition than on the mechanics of 600 m 28 ¹ erosion, and published work on erosion is partly 700 m ′ contradictory. Takahashi (2007) found that the 46°18 N concentration of coarse particles in a debris fl ow 750 m 1 km increases with bed slope, and that erosion is ′ 800 m Check dam only possible when the fl ow is undersaturated 46°18 N 19 in coarse particles. Field observations, however, a 19 SRD Sediment 1200 m indicate that erosion occurs mostly during pas- 16 retention dam sage of the granular fl ow front (Berger et al., a Study reach 17' N 2011), and is likely associated with impacts 1600 m 46° 1 / SRD of coarse sediment on the bed. Iverson et al. 9 / 10 º (2011) explored the role of bed properties and Illgraben ′ found a positive correlation between erosional 46°17 N 2400 m 7°37′E7°38′E7°39′E 7°40'100 E km scour depth and bed water content. Debris-fl ow deposition has been related to channel gradient (Cannon, 1989; Fannin and Wise, 2001; Hungr Figure 1. Overview of Illgraben catchment and fan in southeastern Switzerland. Tributary join- ing downstream of check dam (CD10) is inactive due to hydropower dam in headwaters. et al., 2005; Hürlimann et al., 2003), down- Geophones are mounted on CD1, CD9, CD10, CD28, and CD29. Flow stage measurements are taken at CD10 (radar) and CD29 (laser and radar). Study reach is located between CD16 and *E-mail: [email protected]. CD19. Contour interval is 50 m on fan and 400 m for altitudes above 800 m above sea level. © 2011 The Geological Society of America. For permission to copy, contact Copyright Permissions, GSA, or [email protected]. GEOLOGY,Geology, September September 2011; 2011 v. 39; no. 9; p. 827–830; doi:10.1130/G32103.1; 4 fi gures; Data Repository item 2011241. 827 2007). In the 1970s, a series of concrete check t A) Event 11 B) Event 14 u dams were constructed to limit erosion and 7.6334° E 7.6334° E control the channel position on the fan (Lich- tenhahn, 1971). Flow hydrograph and onset data ¹ 46.2938° N 46.2938° N are available from two gauging stations at CD10 20 m 810 m 810 m near the fan apex (Fig. 1; Badoux et al., 2009) 820 and CD29 at the fan toe (McArdell et al., 2007). m 2 m Since 2007 we have monitored the channel bed 815 using TLS in an unconfi ned 300 m study reach 820 between CD16 and 19 (Fig. 1). 2 m m 815 METHODS 0 m 30 m We surveyed the study reach before and after 5.0 debris fl ows using a Trimble GS200 TLS, yield- 830 1.6 7 ing point clouds of ~10 vertices per survey. m 1.2 m 0.8 Data from individual scan positions and subse- 2 m 825 0.4 quent surveys were merged into one coordinate 820 m 820 0.1 system using an iterative closest point matching 830 m –0.1 algorithm (Besl and McKay, 1992). We gridded –0.4 825 the data to a 0.2-m-resolution digital elevation 2 m –0.8 –1.2 model (DEM) and calculated difference models Elevation change (m) 0 m 30 m –1.6 (Fig. 2) from subsequent surveys; this yields –5.0 a conservative estimate for erosion because it 835 includes deposition in the falling limb of the m 830 m fl ow hydrograph (Berger et al., 2011). For each 830 2 m 830 m fl ow, we mapped maximum inundation limits 835 from levees and mudlines along the channel, m and interpolated these to a 0.2-m-resolution 2 m 830 maximum fl ow stage surface. Our estimated 0 m 20 m uncertainty on this surface is ±0.25 m, given Before debris flow After debris flow Maximum flow surface the diffi culties in identifying the mudline in the fi eld due to splashing and poor preservation. Figure 2. Difference digital elevation models (0.2 m cell size). A: For fl ow 11. B: For fl ow 14. The maximum fl ow stage surface is a lower For fl ow details, see Table DR1 (see footnote 1). Background hillshade image represents estimate as the fl ow surface is generally convex pre-event topography. Color scale values indicate surface elevation change during fl ow; ele- vation change <±0.1 m is shown in white due to uncertainty caused by small-scale surface up in cross section. Flow depth was taken as the roughness. Center panels show elevation change in selected cross sections; black line in- difference between the maximum fl ow stage dicates pre-event topography, red line indicates post-event topography, and blue line indi- surface and the pre-event DEM. We analyzed cates maximum fl ow stage in channel.
Details
-
File Typepdf
-
Upload Time-
-
Content LanguagesEnglish
-
Upload UserAnonymous/Not logged-in
-
File Pages4 Page
-
File Size-