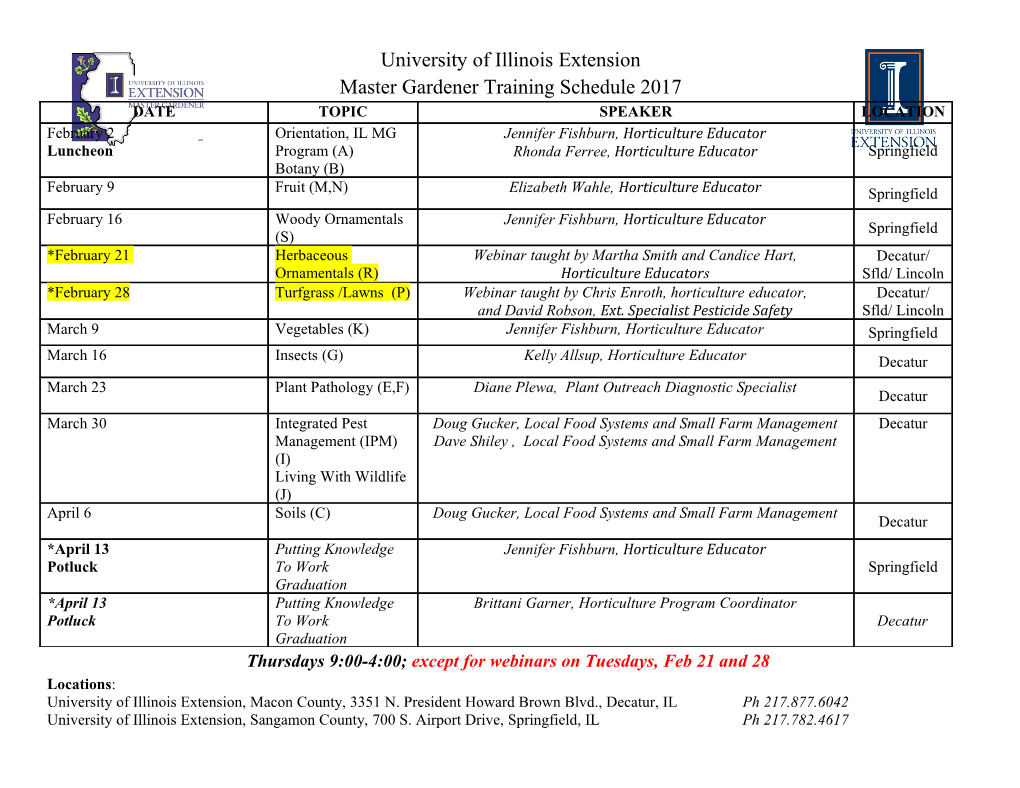
INTERNATIONAL JOURNAL OF MultiCraft International Journal of Engineering, Science and Technology ENGINEERING, Vol. 8, No. 4, 2016, pp. 8-15 SCIENCE AND TECHNOLOGY www.ijest-ng.com www.ajol.info/index.php/ijest 2016 MultiCraft Limited. All rights reserved Generalized model for predicting methane conversion to syngas in membrane reactor Rachna Sinha, Garima Chauhan* University School of Chemical Technology, Guru Gobind Singh Indraprastha University, New Delhi-110078, INDIA *Corresponding Author: e-mail: (Garima Chauhan) [email protected] Abstract Present work aims to provide a conceptual framework for predicting methane conversion efficiency and CO selectivity in a membrane reactor which may assist in selecting the type of membrane and minimizing the cost of syngas production. A comparative evaluation of the performance of ceramic (inorganic) membrane reactors was carried out with other types of synthetic membrane reactors. Data has been taken only from those research papers in which reactor is operating in a temperature range of 800 – 900ºC and the pressure range is 1-2 atm. Linear regression analysis was performed and a generalized equation was developed to predict the methane conversion and CO selectivity using different types of membranes. Simulations were performed using MATLAB ODE45 to investigate feasibility of the developed model. CO selectivity was observed better for ceramic membrane reactors since it achieves 90% saturation in 25 hours while for other synthetic membranes, the conversion is only 10% (approx). Overall, ceramic membrane reactors were found to be better than other synthetic membrane reactor due to effective CO selectivity and CH4 conversion. Keywords: Syngas, CH4 conversion, CO selectivity, ceramic membrane DOI: http://dx.doi.org/10.4314/ijest.v8i4.2 1. Introduction Rapid innovations in technologies and fast paced economic development across the globe are foretokens to edify the necessity of sustainable energy resources. Annual Energy Outlook 2012 projected the increase in world energy consumption by 47% from year 2010 to year 2035 (Energy Outlook, 2012). Global population is projected to reach approximately 8.8 billion people by year 2035 and consequently, world GDP is expected to more than double over the same time period (BP Outlook, 2016). Developing countries China and India are expected to drive the world economy by contributing together for almost 50% of the hike in global GDP (BP Outlook, 2016). Though, economic development and technology advancement are requisite for human welfare, it is pragmatically impossible to have economic growth without concomitant consumption of energy resources (Chauhan et al., 2013). Therefore, an urgent need is felt to look for sustainable energy resources which can be exploited as primary energy source. Fossil fuels are being considered significant contributor in global energy supply, however tremendous increase in greenhouse gas emission and onset of climate change are prevalent issues associated with fossil fuel combustion. Several research efforts are being made worldwide to develop novel technologies for the efficient utilization of renewable energy sources and sequestration of emitted greenhouse gases. Natural gas is being considered the most economically accessible energy resource in present scenario. The ascertained reserves of natural gas amounted world-wide to over 6973 trillion cubic feet (International Energy Statistics, 2015). Call for natural gas is going up by 1.8% per annum which clearly indicates urgent necessity of this fossil fuel. Emerging economies (China and India) account for approximately 30% of the increase in natural gas demand for industrial utilization and power generation (BP Outlook, 2016). Extensive research efforts have been riveted on up-gradation of natural gas to value-added products. Conversion of natural gas to liquid fuels (GTL) through a syngas intermediate (CO/H2) is one of the major research streams in order to conserve the energy 9 Sinha and Chauhan / International Journal of Engineering, Science and Technology, Vol. 8, No. 4, 2016, pp. 8-15 resources. Three possible pathways (steam Reforming, dry reforming and partial oxidation of methane) may be adopted for the natural gas to liquid fuel conversion during GTL process. Steam reforming process (CH4 + steam → CO + H2), being an endothermic process, requires extensive energy supply. Also, poor selectivity for CO and high H2/CO product ratio are the major limitations which make it unsuitable for industrial applications. Dry reforming process (CH4 + CO2 → CO + H2) utilizes the carbon dioxide gas and thus can reduce the greenhouse gas emission, however thermodynamic constraints associated with the process limit conversion efficiency and increases the economics of the process (Gallucci et al., 2008). Partial oxidation of methane (POM) (CH4 + O2 → CO + H2) over the supported transition metals is gaining attention these days due to higher reaction rate, more favourable H2/CO ratio and mild exo-thermicity of the reaction (Wei et al., 2011). Several studies have been reported to identify novel catalysts for syngas production using POM pathway (Sharifnia and Karami, 2015; Wei et al., 2016), however, most of the catalysts for syngas synthesis are active at high temperatures (> 973 K). Secondly, consumption of significant quantities of expensive pure oxygen during POM and the cost associated with the production of pure O2 using cryogenic air separators are added limitations which affect economics of the process. Aforementioned limitations associated with available technologies elucidate the need of an innovative sustainable process for syngas production. Membrane reactors with oxygen semipermeable ionic (or mixed) conducting dense ceramic membranes have drawn considerable attention in last few decades due to their ability to carry out simultaneous reaction and separation (Tong et al., 2006; Silva et al., 2015; Rui et al., 2008). Broadly categorizing, the available membrane reactors can be divided under two categories i.e. ceramic (inorganic) membrane reactors and Organic/metallic/liquid membrane reactors. In the present study, all other synthetically prepared membranes except ceramic membranes are denoted as synthetic membrane reactor. Table 1 provides a brief review about the operating parameters and membrane properties employed in synthetic and ceramic membrane reactors. Table 1 : Literature review on ceramic and synthetic membrane reactor Reactor Membrane Operating Parameter Efficiency References Ceramic Membrane Reactors Dual-phase composite 960°C Time dependence of oxygen Zhu et al., 2010 membrane reactors. permeation flux was observed during initial stages Dense BaCo0.7Fe0.2Ta0.1O3−d 900°C CH4 conversion > 99% ; CO Luo et al., 2010 perovskite membrane selectivity : 94% mixed conducting ceramic operating time : 2200 h CH4 conversion > 96% ; CO Tong et al., 2006 membrane reactors. Temp: 900°C selectivity > 95% Pressure: 1-1.2atm Hollow fibre catalytic reactor, perovskite mixed conducting 925°C CO selectivity > 97% ; CH4 Wang et al., 2006 Ni-based catalyst membrane (BaCoxFeyZrzO3-d ) conversion : 96%. Membrane Reactor ceramic composite of Ba0.5Sr0.5 T : 900°C No significant change in CO Chen et al., 2003 Co0.8Fe0.2O 3_d (97.5 mol%) and Pressure : 1 atm selectivity with variation of CH4 Co3O4 (2.5 mol%). feeding rate Tubular reactor (Catalyst : a perovskite material Temperature: 875°C CO selectivity > 95% ; CH4 Wang et al., 2003 LiLaNiO/–Al2O3) Ba0.5Sr0.5Co0.8Fe0.2O3−δ , with Conversion > 94% electronic and ionic conductivity. Catalytic membrane reactor, zirconium-based dense membrane, Temperature : 850°C CH4 conversion : 96–98%; CO Tong et al., 2002 LiLaNiO/γ -Al2O3 catalyst with mixed conducting perovskite selectivity : 98–99% 10wt.% nickel loading (BaCo0.4Fe0.4Zr0.2O3−δ) Catalyst packed tubular reactor Perovskite-type oxide Temperature : 1098- CH4 conversion > 96% ; CO Jin et al., 2000 La0.6Sr0.4Co0.2Fe0.8O3-d 1158 K selectivity > 97% at low carbon space velocity Other Synthetic Membrane Reactors Fixed bed reactor, Ni-based Pd-Ag membrane Temp : 823K, 873 K Hydrogen yield reached 21% at 823 Silva et al., 2015 catalyst Pressure : 1.01×105 Pa K and 47% at 873K Tubular membrane reactors Pd–Ag alloy membrane Temperature ranges 350 Maximum CO2 conversion : 20.6% Gallucci et al., 2008 -450°C. for the MR at 450°C, 5 10 Pa maximum CH4 conversion: 17.41% for the TR at 450°C Tubular reactor, Ni/γ-Al2O3 oxygen-permeable SrFeCo0.5Oy Temperature : 900 °C; Throughput conversion of CH4 : 98%, Fend et al., 2004 catalyst membrane CH4 feeding rate : 26.8 CO selectivity : 98%, H2/CO : 1.8, mL/min syngas generation rate : 16 mL/min/cm2 a conventional reactor (CR) 50μm thick pinhole free Pd-Ag Maximum CH4 conversion : 69% at Gallucci et al., 2004 and a membrane reactor (MR) alloy membrane 450°C a traditional reactor, Membrane composite ceramic palladium Temperature : 250– DPMR showed highest methane Basile and Paturzo, reactor, Ni-based catalyst membrane reactors (CPMR), and a 550°C. conversion: 2001 dense palladium membrane reactor 45.3% at 400°C and 56.2% at 450°C. (DPMR) Traditional and membrane Palladium based membrane Time factor of 4288 gcat Maximum CH4 conversion: 96% Basile et al., 2001 reactor min / mol, p = 1.2 bar using palladium membrane reactor at 550°C 10 Sinha and Chauhan / International Journal of Engineering, Science and Technology, Vol. 8, No. 4, 2016, pp. 8-15 Present study compares a variety of membranes for the conversion of CH4 to syngas. An extensive literature survey has
Details
-
File Typepdf
-
Upload Time-
-
Content LanguagesEnglish
-
Upload UserAnonymous/Not logged-in
-
File Pages8 Page
-
File Size-