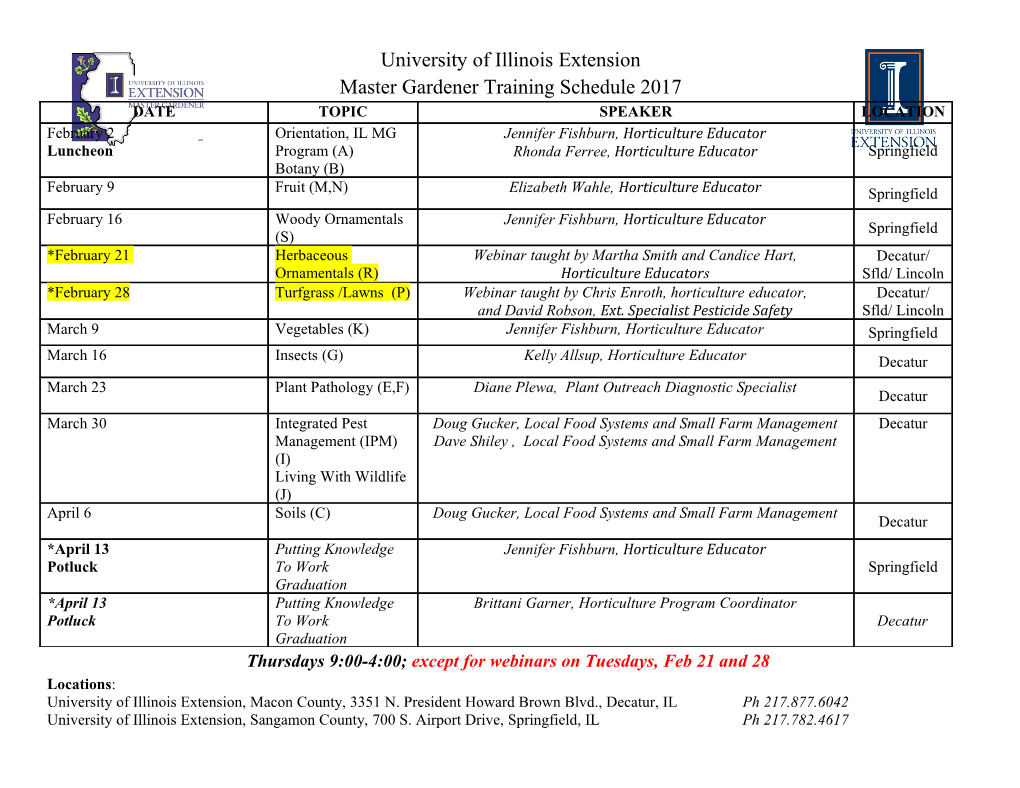
Copyright 1999 by the Genetics Society of America Characterization of the Repeat-Tract Instability and Mutator Phenotypes Conferred by a Tn3 Insertion in RFC1, the Large Subunit of the Yeast Clamp Loader Yali Xie,* Chris Counter² and Eric Alani* *Section of Genetics and Development, Cornell University, Ithaca, New York 14853-2703 and ²Department of Pharmacology and Cancer Biology, Department of Radiation Oncology, Duke University Medical Center, Durham, North Carolina 27710 Manuscript received August 3, 1998 Accepted for publication October 22, 1998 ABSTRACT The RFC1 gene encodes the large subunit of the yeast clamp loader (RFC) that is a component of eukaryotic DNA polymerase holoenzymes. We identi®ed a mutant allele of RFC1 (rfc1::Tn3) from a large collection of Saccharomyces cerevisiae mutants that were inviable when present in a rad52 null mutation background. Analysis of rfc1::Tn3 strains indicated that they displayed both a mutator and repeat-tract instability phenotype. Strains bearing this allele were characterized in combination with mismatch repair (msh2D, pms1D), double-strand break repair (rad52), and DNA replication (pol3-01, pol30-52, rth1D/rad27D) mutations in both forward mutation and repeat-tract instability assays. This analysis indicated that the rfc1::Tn3 allele displays synthetic lethality with pol30, pol3, and rad27 mutations. Measurement of forward mutation frequencies in msh2D rfc1:Tn3 and pms1D rfc1:Tn3 strains indicated that the rfc1::Tn3 mutant displayed a mutation frequency that appeared nearly multiplicative with the mutation frequency exhibited by mismatch-repair mutants. In repeat-tract instability assays, however, the rfc1::Tn3 mutant displayed a tract instability phenotype that appeared epistatic to the phenotype displayed by mismatch-repair mutants. From these data we propose that defects in clamp loader function result in DNA replication errors, a subset of which are acted upon by the mismatch-repair system. UTATIONS in genes that are involved in DNA A major type of chromosomal instability that has been M replication and repair often result in chromo- identi®ed in yeast, in bacteria, and in cancer cells is somal instabilities such as base pair substitutions and repeat-tract instability (reviewed in Crouse 1996; Kolod- frameshifts as well as insertion, deletion, and re- ner 1996; Modrich and Lahue 1996; Sia et al. 1997b). arrangement events (i.e., Schaaper and Dunn 1987; This instability is thought to result mainly from the Aguilera and Klein 1988; Strand et al. 1993; McAlear failure to repair DNA slippage events that occur during et al. 1996; Tran et al. 1996; Green and Jinks-Robert- the replication of repetitive DNA sequences such as son 1997). Mutations that confer these phenotypes have those that contain mono-, di-, tri-, and tetranucleotide been directly identi®ed in Escherichia coli and Saccharo- repeats. In both yeast and mammalian cells, mutations myces cerevisiae using a variety of chromosome instability in mutS and mutL homolog mismatch-repair genes result assays (i.e., Feinstein and Low 1986; Aguilera and in a large increase (100- to 10,000-fold) in the rate of Klein 1988; Michaels et al. 1990; Jeyaprakash et al. repeat-tract instability (Strand et al. 1993; Tran et al. 1994). Such basic research approaches have also led to 1997; Umar et al. 1998). This increase is thought to be a better understanding of the underlying chromosome due to the inability of these mutants to repair small stability defects that have been observed in patients who loop insertion/deletion mutations that occur during suffer from particular inherited diseases. For example, DNA slippage (reviewed in Crouse 1996; Kolodner the phenotypes exhibited by mismatch-repair-defective 1996; Modrich and Lahue 1996; Sia et al. 1997b). In E. coli and S. cerevisiae strains provided evidence indi- yeast, mutations in DNA replication genes that encode cating that the underlying cause for a large percentage the polymerase processivity factor PCNA (POL30) and of hereditary nonpolyposis colorectal cancers was a de- the ¯ap endonuclease (RTH1/RAD27) have also been fect in mismatch repair (Levinson and Gutman 1987; shown to confer an increase in repeat-tract instability at Strand et al. 1993; Crouse 1996; reviewed in Kolodner a rate that is similar to that observed in mismatch-repair- 1996; Modrich and Lahue 1996). defective mutants (Johnson et al. 1995, 1996; Umar et al. 1996; Kokoska et al. 1998). Mutations in other DNA replication genes, such as those that encode the Pold (POL3), and Polε (POL2) DNA polymerases, however, Corresponding author: Eric Alani, Section of Genetics and Develop- ment, Cornell University, 459 Biotechnology Bldg., Ithaca, NY 14853- resulted in only modest increases in repeat-tract instabil- 2703. E-mail: [email protected] ity (Johnson et al. 1995; Tran et al. 1997; Kokoska et Genetics 151: 499±509 (February 1999) 500 Y. Xie, C. Counter and E. Alani al. 1998). These data have led to the proposal that some man 1989; Burgers 1991; Fien and Stillman 1992; DNA replication factors function directly in the mis- Stillman 1994). match-repair pathway to repair loop insertion/deletions that result from DNA slippage events while others act In this study we tested strains bearing the rfc1::Tn3 at the level of DNA replication to prevent the formation allele alone or in combination with mismatch repair and of these events (Kokoska et al. 1998; reviewed in Sia et DNA replication mutations for defects in chromosome al. 1997b). stability. As described below, the rfc1::Tn3 mutation con- The above observations, in conjunction with the obser- ferred a mutator phenotype that appeared multipli- vation that many cancer cells display repeat-tract insta- cative with mismatch-repair mutations. In repeat-tract bilities that are unlinked to previously identi®ed repair stability assays the rfc1::Tn3 mutation conferred an z10- and replication genes, encouraged us to initiate screens fold increase in the frequency of dinucleotide repeat- in yeast to identify chromosomal instability mutants tract instability that appeared to be epistatic to the phe- (Liu et al. 1995). We hoped to identify additional factors notype observed in mismatch-repair-defective mutants. that are involved in preventing mutagenic replication Taken together, our data are consistent with the idea errors by preventing DNA slippage events or by facilitat- that defects in clamp loader function result in DNA ing the repair of these slippages through mismatch- replication errors, a subset of which are identi®ed and repaired by the mismatch-repair system. repair mechanisms. In one screen we searched for mu- tants that displayed an increase in the frequency of both repeat-tract insertion/deletion and base pair substitu- MATERIALS AND METHODS tion/frameshift events; unfortunately, this screen only identi®ed mutants that displayed mutations in the pre- Media and chemicals: E. coli strains were grown in Luria- viously characterized mutS (MSH2) and mutL (PMS1, Bertani (LB) broth or on LB agar, which was supplemented m MLH1) homolog genes and the RAD27 gene (Y. Xie, with 100 g/ml ampicillin when required (Miller 1972). Yeast strains were grown in either YPD or minimal selective L. Schvaneveldt and E. Alani, unpublished data). In media (Rose et al. 1990). Selective media contained 0.7% yeast a second screen that is the focus of this article, we nitrogen base, 2% agar, 2% glucose, and 0.09% of a drop-out searched for mutants that were inviable in a double- mix that lacks the amino acid used for selection. Sporulation strand break-repair-de®cient (rad52) background and media (SPM) were prepared as described previously (Detloff also displayed a mutator phenotype (Counter et al. et al. 1991). When required, canavanine was included in mini- mal selective media at 60 mg/liter (Rose et al. 1990). 5-¯uoro- 1997). This screen was conducted on the basis of two orotic acid (5-FOA) was purchased from U.S. Biologicals and observations made in E. coli: used as described previously (Boeke et al. 1984). When re- quired, methyl-methane sulfonate (MMS; Aldrich Chemical, 1. Certain DNA replication mutants display chromo- Milwaukee) was included in YPD media at 0.017% (v/v). some instability defects such as chromosomal break- E. coli strains: DH5a [F9 phi80, dLacZD (lacZYA-argF), U169, 2 1 2 ages that are lethal in recombination-de®cient back- recA1, endA1, hsdr17 (r K, m K), lambda , thi1, gyrA, relA1] and KC8 (gammax14862, MK121, leuB600, trpC9830, pyrF::Tn5, grounds (Michel et al. 1997). hisB463, Dlacx74, StrA, galU, K) were used to amplify and 2. Strains that lack dam methylase, and are thus defec- manipulate all plasmids described in this article. tive in strand discrimination during mismatch re- S. cerevisiae strains: The genotypes of all strains used in pair, display a mutator phenotype and are inviable these studies are shown in Table 1. With the exception of in recombination de®cient (recA2) backgrounds NKY1068, EAY561, and DNR53, all strains were derived from the isogenic FY strain background (Winston et al. 1995). (McGraw and Marinus 1980). This inviability, DNR53 is also an S288C-derived strain that contains the which can be rescued by mutations in the mutS, rfc1::Tn3 allele (Burns et al. 1994; Counter et al. 1997). This mutL, and mutH mismatch-repair genes, is thought strain also contains a rad52::HIS3 mutation and is viable be- to be caused by unrepairable double-strand breaks cause it contains an ARS1, CEN4, URA3, plasmid bearing the in dam2 recA2 strains that form as the result
Details
-
File Typepdf
-
Upload Time-
-
Content LanguagesEnglish
-
Upload UserAnonymous/Not logged-in
-
File Pages11 Page
-
File Size-