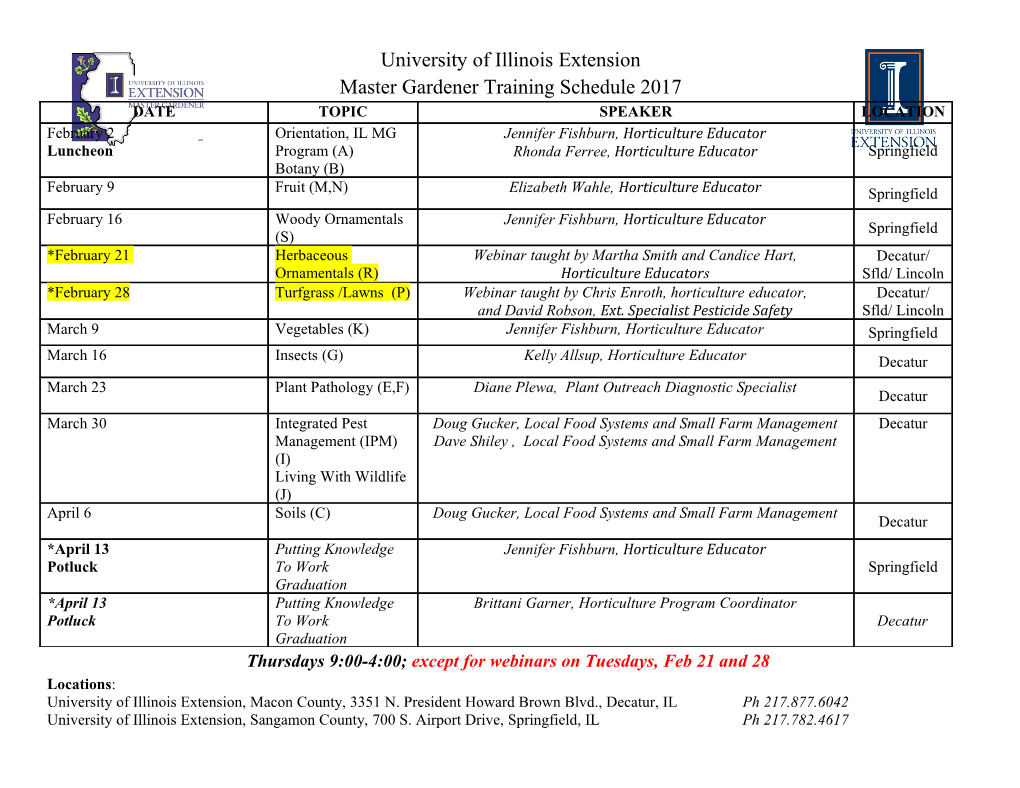
This thesis has been approved by The Honors Tutorial College and the Department of Communication Sciences & Disorders Dr. Li Xu Professor, Communication Sciences & Disorders Thesis Advisor Dr. Chao-Yang Lee Professor, Communication Sciences & Disorders Director of Studies, Communication Sciences & Disorders Dr. Donal Skinner Dean, Honors Tutorial College 2 PERCEPTION OF SPECTRALLY-DEGRADED, FOREIGN-ACCENTED SPEECH A Thesis Presented to The Honors Tutorial College Ohio University In Partial Fulfillment of the Requirements for Graduation from the Honors Tutorial College with the degree of Bachelor of Science in Communication Sciences & Disorders By Jenna Barrett April 2021 3 Introduction Background on Speech Perception Speech perception is an incredible and complicated process that allows us to hear, interpret, and understand speech sounds. It begins with a speech signal. Most of us understand speech as a string of letters which make up words, which make up sentences, which go on to form even larger elements of language. Physically, however, speech is an acoustic wave. This complex wave is made up of numerous sounds that run together and occasionally overlap, just as they do in words and sentences. The smallest units of sound are known as phonemes. When an acoustic wave reaches the ear, vibrations pass through the hearing structures and stimulate the auditory nerve. These nerve impulses are processed by the brain and integrated with previous knowledge of sound to allow for the comprehension of speech (Urbas, 2019). Within the nervous system, there are specialized mechanisms for processing speech. This is evidenced by experiments demonstrating that phonemes can be perceived at rates seven times faster than that of nonspeech sounds. What is perhaps even more impressive is the flexibility of this system. Humans can easily understand speech in their native language, often regardless of speech rate, voice, pitch, accent, loudness, or signal distortion. Even though the acoustic signal may be changed or distorted in many of these cases, the nervous system is able to rapidly adjust and compensate for these variations. This is known as perceptual constancy (Urbas, 2019). There are two main types of speech processing theories involved in perception: bottom-up processing and top-down processing (Figure 1). Bottom-up processing refers to using the simplest elements of an acoustic wave to guide more complex perception. 4 Within the wave, there are acoustic cues to phonemes, which can be combined into larger and larger speech components. Top-down processing is essentially the opposite. Complex linguistic knowledge, including context clues and sentence predictability, are used to anticipate speech. Both types of processing are necessary for speech perception. For example, in one study, researchers presented participants with a pair of words where the initial sound was a mixture of the /d/ and /t/ American English phonemes. In one instance, the phoneme made a nonsense word (drash) and in the other instance, the phoneme made a real word (trash). Participants were more likely to perceive the phoneme that created a real word (Austermann and Yamada, 2009). This is an example of the combination of processing techniques. Bottom-up processing would inform participants that the acoustic signal contained a phoneme similar to either /d/ or /t/, but top-down processing would allow participants to use previous linguistic knowledge to choose the phoneme that produced a real word. Figure 1. The figure above describes the interaction between top-down and bottom-up processing in the perception of a speech stimulus. The basic information derived from the acoustic wave as well as higher-level contextual information are important for speech perception (Austermann and Yamada, 2009). 5 In research based on non-tonal languages, it was proposed that bottom-up and top-down processing occurred in series, with application of the acoustic signal coming before the use of more complex linguistic knowledge. However, in recent research on tonal languages, it was found that both types of processing were present in early and late stages of processing. Researchers used event-related potentials (ERPs) to track the use of bottom-up and top-down processing in lexical tone perception. Based on the results, a parallel lexical tone processing model was proposed (Shuai and Gong, 2014). This research raises questions as to the different types of processing that may occur in native speakers of a language (L1) and second language learners (L2) when presented with speech that contains acoustic-phonetic deviations. The Normal Hearing Mechanism The hearing mechanism is an integral component of speech perception. In a normal hearing individual, the hearing process begins with sound. When an object vibrates, the pressure causes particles in the surrounding medium to move, creating a sound wave. This sound wave is funneled into the ear canal, causing the tympanic membrane to vibrate. In the middle ear, these vibrations are passed to three small ossicles known as the malleus, incus, and stapes. These bones vibrate in sequence and function to correct the impedance mismatch, or loss of sound energy, that occurs when traveling from an air to fluid medium. The mechanical vibrations from the ossicles are then transferred to the fluid-filled cochlea of the inner ear. The cochlea contains three membranes: Reissner’s membrane, the basilar membrane, and the tectorial membrane. The basilar membrane is organized tonotopically, meaning different areas of the 6 membrane respond to different frequencies. In the case of the basilar membrane, the basal portion is stimulated by higher frequencies and the apical portion is stimulated by lower frequencies. On the basilar membrane lies the organ of Corti, which contains sensory hair cells of hearing. Ultimately, the inner hair cells are responsible for the neural impulse that travels to the brain. There are three fluid-filled passages within the cochlea containing either perilymph or endolymph. Perilymph fills the scala vestibuli and scala tympani, the two chambers separated by the basilar membrane. Perilymph is rich in sodium ions. In contrast, endolymph fills the scala media, which is the chamber between the scala vestibuli and scala tympani, also known as the cochlear duct. Endolymph is rich in potassium ions. Inside the organ of Corti, inner hair cells are grouped in a single row, and each hair cell is linked to about ten nerve cells. These inner hair cells consist of a main body and thin stereocilia that stick out of the basilar membrane. Fluid vibrations within the cochlea cause stereocilia of hair cells in specific portions of the basilar membrane to bend. This bending opens up ion channels between the stereocilia and hair cells, altering the concentration of sodium and potassium ions in the nerve cell. In response, potassium rushes into the hair cells, depolarization of the hair cell occurs, and neurotransmitters (glutamate) are released from the bottom of the hair cells. The auditory nerves pick up the neurotransmitters and generate action potentials (Niparko, 2000). These electrical impulses are transmitted to the brain via the auditory nerve. The diagram below (Figure 2) illustrates this hearing process up to the cochlear level. 7 Figure 2. The figure above shows the anatomical structures involved in the mechanism of hearing. Sound waves enter the ear canal and cause the tympanic membrane to vibrate. These vibrations are amplified by the ossicles of the middle ear and transferred to the inner ear. Fluid vibrations in the cochlea cause hair cells to bend, creating electric potentials that stimulate the auditory nerve and create the perception of sound (Hawkins, 2020). Beyond the cochlea, the neural impulse must be transmitted from the cochlear nerve to the auditory cortex for identification and perception. Information from the cochlea follows the auditory pathway, which consists of roughly 4 sequential areas in the brain that work to decode the signal. The pathway begins with the cochleo-vestibular nerve. Recall that electrical impulses travel here via the auditory nerves that synapse with the hair cells in the cochlea. From the cochleo-vestibular nerve, the impulses travel to the cochlear nuclei of the brain stem where information on duration, intensity, and frequency of the signal is deciphered. Next, a neuron carries this information to the superior olivary complex of the brainstem. It is important to note that, while both brain hemispheres process sound stimuli from both ears, much of the information from the stimuli passes to the contralateral side at this junction. The signal then travels to the inferior colliculus of the brainstem. Both the superior olivary complex and inferior colliculus work to integrate information from both ears and determine sound lateralization and localization. A fourth 8 neuron then carries the signal up to the medial geniculate body of the thalamus, which functions to focus auditory attention and prepare a response to the sound signal. The last stop is the auditory cortex in the temporal lobe; here, the message of the signal is received and translated. Similar to the basilar membrane, the auditory cortex is organized tonotopically, with certain neurons tuned to specific frequencies; this allows for time and frequency information to be decoded. In addition, the primary auditory cortex is involved in identifying the sound, employing memory, and, if necessary, generating a response (Pujol, 2020). Information from the
Details
-
File Typepdf
-
Upload Time-
-
Content LanguagesEnglish
-
Upload UserAnonymous/Not logged-in
-
File Pages61 Page
-
File Size-