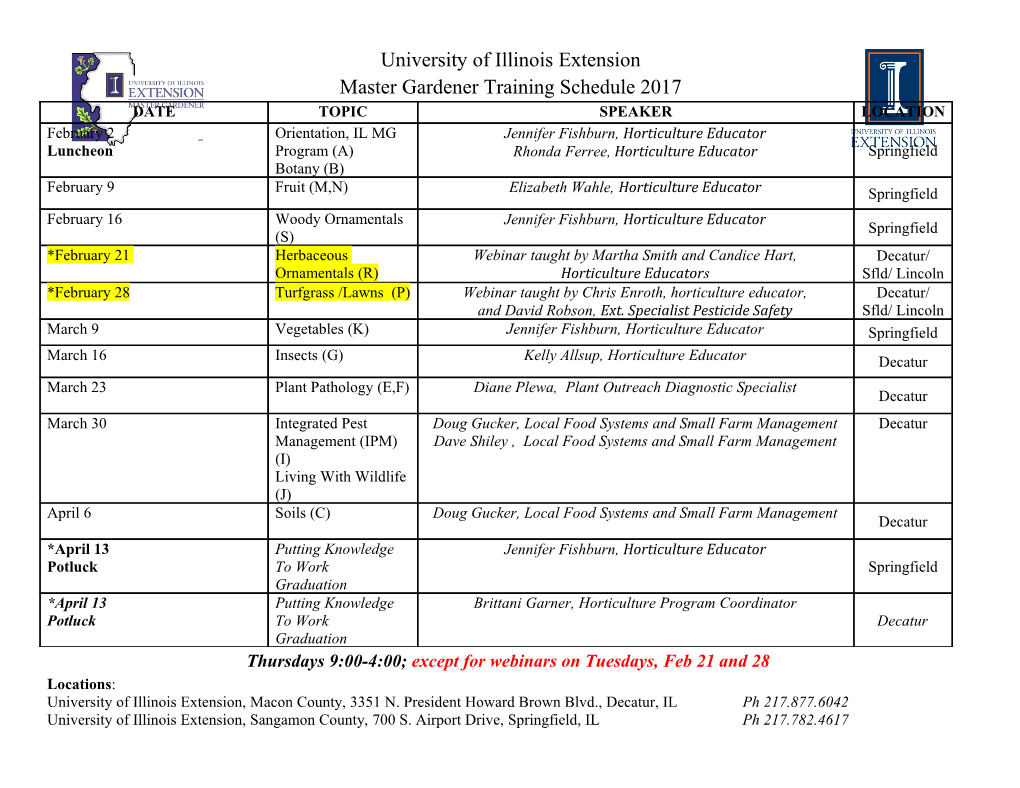
UNIVERSITY OF CALIFORNIA, IRVINE Kinetic and Thermodynamic Factors for Selective CO2 Reduction at a Metal Hydride DISSERTATION submitted in partial satisfaction of the requirements for the degree of DOCTOR OF PHILOSOPHY in Chemistry by Bianca Myriah Ceballos Dissertation Committee: Associate Professor Jenny Y. Yang, Chair Professor A.S. Borovik Professor Alan F. Heyduk 2019 Portion of Chapter 1 © 2017 The Royal Society of Chemistry Portion of Chapter 2 © 2018 National Academy of Sciences DEDICATION Para Virginia & Angela. mi abuelas. quien soñaban con una vida mejor un sol naciente For Olivia & Martin. my parents. who lit a light of curiosity within me a warm fire For Justine. my sister. my hero. a sunrise summit For Erick. my brother. a bubbling brook overflowing For Sean. my youngest brother. a bird’s song For Zach. my cheerleader. falling snow For Rocky. my warm hug. an earthbound star ii TABLE OF CONTENTS Page LIST OF FIGURES v LIST OF TABLES xi LIST OF SCHEMES xii LIST OF CHARTS xiii ACKNOWLEDGEMENTS xiv CURRICULUM VITAE xvi ABSTRACT OF THE DISSERTATION xxi INTRODUCTION 1 0.1 Energy Storage in Chemical Bonds 2 0.2 Electrochemical CO2 Reduction Strategies 3 0.3 Metal Hydricity in Catalysis 6 0.4 Research Goals 8 0.5 References 11 CHAPTER 1: Hydricity Determination, Solvent Effects, and Reactivity of 20 + [Ni(tmepe)2] 1.1 Motivations and Specific Aims 21 1.2 Background 21 1.3 Results and Discussion 24 1.3.1 Synthesis and Structure of [Ni(TMEPE)2][BF4]2, 24 [HNi(TMEPE)2][BF4], and [Ni(TMEPE)2] (TMEPE = 1,2- bis[di(methoxyethyl)phosphino]ethane) 1.3.2 Benchmarking Metal Hydricity Values in Acetonitrile, 25 Dimethylsulfoxide (DMSO), and Water + 1.3.3 Reactivity of [HNi(TMEPE)2] towards CO2 reduction and formate 28 - (HCO2 ) oxidation reactions 1.4 Conclusion 33 1.5 Experimental Details 34 1.6 References 46 + CHAPTER 2: Selective CO2 Reduction at [HPt(dmpe)2] through 56 Thermodynamic and Kinetic Control 2.1 Motivations and Specific Aims 57 2.2 Background 57 iii 2.3 Results and Discussion 60 2.3.1 Constructing Thermodynamic Product Diagrams 60 2.3.2 Application of Thermodynamic Product Diagrams for Catalyst 64 Discovery 2.3.3 Selective Electrocatalytic Reduction of CO2 to Formate 68 2.3.4 Kinetics of Electron Transfer 74 2.3.5 Reactivity of [Pt(dmpe)2] with CO2 80 + 2.3.6 Reactivity of [Pt(dmpe)2] with H 84 2.3.7 Kinetic Control vs. Thermodynamic Control 85 2.3.8 Applicability of Thermodynamic Product Diagrams 87 2.4 Conclusion 90 2.5 Experimental Details 93 2.6 References 98 APPENDIX A: Electrochemical Investigation of Al(OTf)3 Cation Effects on 109 FeTPPCl and FeF20TPPCl CO2 Reduction Reactivity A.1 Motivations and Specific Aims 110 A.2 Background 110 A.3 Results and Discussion 112 A.3.1 FeF20TPPCl Titration with Mg(ClO4)2 in DMF 112 A.3.2 FeF20TPPCl with Mg(ClO4)2 under CO2 in DMF 114 A.3.3 FeF20TPPCl with Mg(ClO4)2 under CO2 with H2O in DMF 115 A.3.4 FeF20TPPCl and FeTPPCl Titration with Al(OTf)3 in DMF 117 A.3.5 FeTPPCl with Al(OTf)3 under CO2 with H2O in DMF 119 A.3.6 FeF20TPPCl with Al(OTf)3 under CO2 with H2O or phenol in DMF 121 A.3.7. Al(OTf)3 under CO2 with H2O in DMF 122 A.4 Conclusions 124 A.5 Experimental Details 125 A.6 References 126 iv LIST OF FIGURES Page Figure 0.1 Plot of energy density by mass and volume for Li-ion batteries and 2 chemical fuels. Figure 1.1 Structural representation (left) and solid-state structure (right) of 25 [Ni(TMEPE)2][BF4]2 (2). Crystal structure details: asymmetric unit contains half of the molecule, other half generated by inversion around Ni; only one orientation of a disordered methoxyethyl group shown; thermal ellipsoids drawn at 50%; hydrogen atoms and two BF4 anions omitted for clarity. Figure 1.2 31P{1H} NMR spectrum showing complete lithium formate oxidation 29 by complex 2 in DMSO-d6 to generate complex 1 after the addition of 1 eq of lithium formate, under N2. Figure 1.3 31P{1H} NMR spectrum showing lithium formate oxidation by complex 30 2 (*) in CD3CN to generate complex 1 (o) after the addition of 2 eq of lithium formate under N2. Figure 1.4 31P{1H} NMR spectrum of no reaction between complex 2 (*) and one 30 equivalent of lithium formate in D2O under CO2. Figure 1.5 31P{1H} NMR spectrum showing nickel hydride complex 3 (o) in pH 9 31 carbonate buffered D2O with background protonation to complex 2 (*). Complex 1 generated from protonation of Ni(0) complex 3 by [PhNH4][BF4] in CD3CN. Figure 1.6 31P{1H} NMR spectrum showing stability of nickel hydride complex 1 32 (o) in pH 10.4 carbonate buffered H2O under N2. Figure 1.7 31P{1H} NMR spectrum showing nickel hydride complex 1 in pH 9 33 buffered H2O under N2 (top), nickel hydride complex 1 at pH 7 after addition of CO2 (middle), nickel hydride complex 1 at pH 7 after addition of O2. Where complex 1 is denoted by (o), complex 2 is denoted by (*), and unidentified decomposition complex is denoted by (X). Figure 1.8 1H NMR spectrum of tmepe ligand (tmepe = 1,2- 39 bis[di(methoxyethyl)phosphino]ethane) in CDCl3 where the black X indicate unknown impurity peaks. Figure 1.9 31P{1H} NMR spectrum of tmepe ligand (tmepe = 1,2- 40 bis[di(methoxyethyl)phosphino]ethane) in CDCl3 where the black X denotes impurity peaks. v 1 Figure 1.10 H NMR spectra of [Ni(tmepe)2][BF4]2 in CDCl3. 40 31 1 Figure 1.11 P{ H} NMR spectra of [Ni(tmepe)2][BF4]2 in CDCl3. 41 1 Figure 1.12 H NMR spectrum of Ni(tmepe)2 (3) in CD3CN. 41 31 1 Figure 1.13 P{ H} NMR spectrum of Ni(tmepe)2 (3) in CD3CN. 42 Figure 1.14 1H NMR spectrum showing the protonation of one equivalent of the 42 Ni(0) complex 3 by the addition of one equivalent of [PhNH4][BF4] in CD3CN to yield the nickel hydride complex 1 (o). Figure 1.15 31P{1H} NMR spectrum showing the protonation of Ni(0) complex 3 to 43 nickel hydride complex 1 in CD3CN by one equivalent of [PhNH4][BF4]. Figure 1.16 1H NMR spectrum showing the protonation of one equivalent of the 43 Ni(0) complex 3 by the addition of one equivalent of [PhNH4][BF4] in DMSO-d6 to yield the nickel hydride complex 1(o), where aniline peaks are denoted by A. Figure 1.17 31P{1H} NMR spectrum showing the protonation of complex 3 to 1 in 44 DMSO-d6 by one equivalent of [PhNH4][BF4]. Figure 1.18 1H NMR spectrum showing nickel hydride complex 1(o) in pH 10 44 carbonate buffered D2O. Figure 1.19 31P{1H} NMR spectrum showing nickel hydride complex 1 (o) in pH 10 45 carbonate buffered D2O. Figure 2.1 Cyclic voltammetry under 1 atm of N2 of 0.5 mM solution of 65 [Pt(dmpe)2](PF6)2 (1) (black); after addition of 1 equivalent of phenol (blue); and 5 equivalents of phenol (green); and under CO2 with 5 equivalents of phenol (red); and 10 equivalents phenol (orange). Conditions: 0.1 M Et4NPF6, 1mM Fe(C5H5)2 present as an internal reference, glassy carbon working and auxiliary electrode, Ag/AgCl vi pseudoreference electrode, 10 mV/s scan rate. 31 1 Figure 2.2 P{ H} NMR spectra of [HPt(dmpe)2](PF6) (top) and after addition 1 66 + equivalent of phenol (bottom). Where ^ denotes [HPt(dmpe)2] and * denotes PF6 ion. Figure 2.3 Cyclic voltammetry of 1 mM [HPt(dmpe)2](PF6) (2) in 0.1 M TBAPF6 67 in CH3CN at 100 mV/s scan rate; under N2. +/0 Figure 2.4 Current vs. Time plot of 1 hour electrolysis at -2.4 V vs. Fe(C5H5)2 of 69 1 mM [Pt(dmpe)2](PF6)2, 10 mM phenol and 1 mM FeCp2 with 0.1 M TBAPF6 in CH3CN; under CO2 (run 1). Total charge passed: 18.2 C; 3.14 equivalents of charge with respect to 3. +/0 Figure 2.5 Current vs. Time plot of 1 hour electrolysis at -2.3 V vs. Fe(C5H5)2 of 69 1 mM [Pt(dmpe)2](PF6)2, 10 mM phenol and 1 mM FeCp2 with 0.1 M TBAPF6 in CH3CN; under CO2 (run 2). Total charge passed: 22.4 C; 3.87 equivalents of charge passed with respect to 3. Figure 2.6 GC calibration curve for H2 with 1 hour mixing time in acetonitrile. 70 Points show average peak area and error bars show the standard deviation across 6 measurements. Linear fit equations are shown on the graph. Figure 2.7 1H NMR post electrolysis solution of run 1 with DMF standard 70 (method A formate quantification). Run 1 electrolysis solution: 10 mM phenol and 1 mM Fe(C5H5)2 with 0.1 M TBAPF6 in CH3CN; under CO2. 68 1 Figure 2.8 H NMR post electrolysis solution of run 1 with DMF standard following solution acidification (method B formic acid quantification). Run 2 electrolysis solution: 10 mM phenol and 1 mM Fe(C5H5)2 with 0.1 M TBAPF6 in CH3CN; under CO2. Figure 2.9 1H NMR solvent suppression calibration curve for formic acid in 71 acetonitrile. Points show peak area Linear fit equations are shown on the graph.
Details
-
File Typepdf
-
Upload Time-
-
Content LanguagesEnglish
-
Upload UserAnonymous/Not logged-in
-
File Pages148 Page
-
File Size-