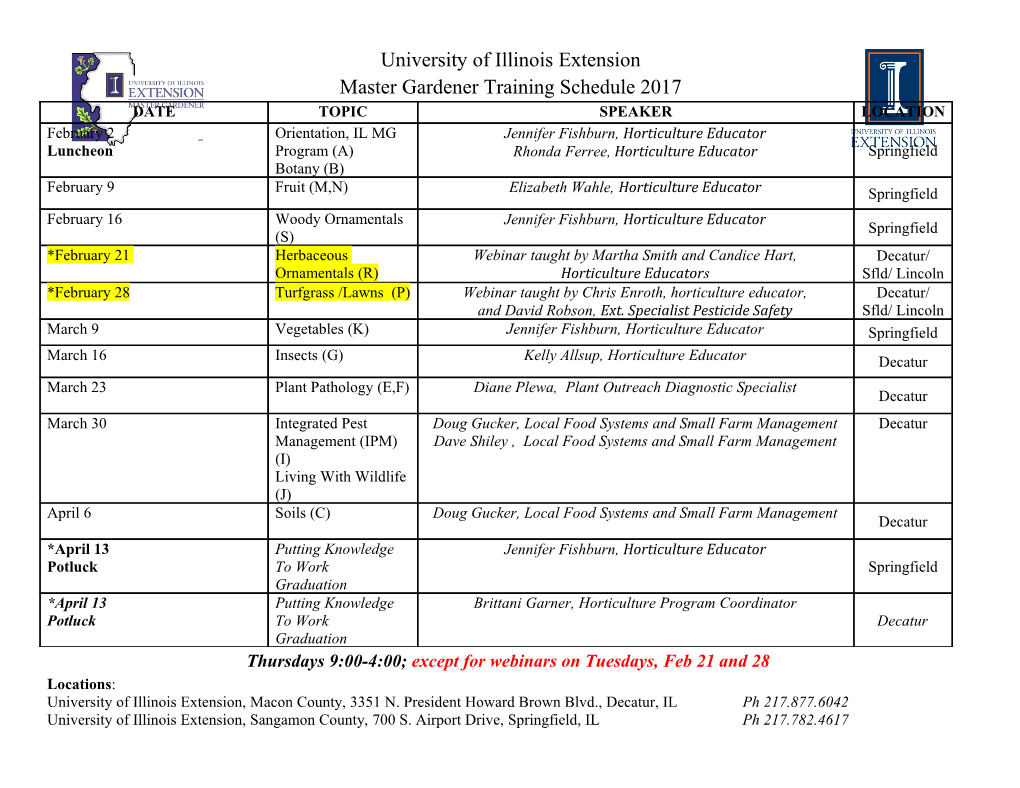
A&A 479, 123–129 (2008) Astronomy DOI: 10.1051/0004-6361:20077956 & c ESO 2008 Astrophysics Active galactic nuclei and massive galaxy cores S. Peirani1,2,S.Kay1,3, and J. Silk1 1 Department of Physics, University of Oxford, Denys Wilkinson Building, Keble Road, Oxford OX1 3RH, UK e-mail: [sp;silk]@astro.ox.ac.uk 2 Institut d’Astrophysique de Paris, 98 bis Bd Arago, 75014 Paris; Unité mixte de recherche 7095 CNRS, Université Pierre et Marie Curie, France 3 Jodrell Bank Centre for Astrophysics, Alan Turing Building, School of Physics and Astronomy, The University of Manchester, Manchester M13 9PL, UK e-mail: [email protected] Received 27 May 2007 / Accepted 30 November 2007 ABSTRACT Context. Central active galactic nuclei (AGN) are supposed to play a key role in the evolution of their host galaxies. In particular, the dynamical and physical properties of the gas core must be affected by the injected energy. Aims. Our aim is to study the effects of an AGN on the dark matter profile and on the central stellar light distribution in massive early type galaxies. Methods. By performing self-consistent N-body simulations, we assume in our analysis that periodic bipolar outbursts from a central AGN can induce harmonic oscillatory motions on both sides of the gas core. Results. Using realistic AGN properties, we find that the motions of the gas core, driven by such feedback processes, can flatten the dark matter and/or stellar profiles after 4−5 Gyr. These results are consistent with recent observational studies that suggest that most giant elliptical galaxies have cores or are “missing light” in their inner part. Since stars behave as a “collisionless” fluid similar to dark matter, the density profile both of stars and dark matter should be affected in a similar way, leading to an effective reduction in the central brightness. Key words. methods: N-body simulations – galaxies: structure – galaxies: active 1. Introduction a similar problem: where has the gas gone? But we stress that there are many ways of accounting for this and other objections The cold dark matter (CDM) paradigm (Cole et al. 2005, (we provide more details below). The point we wish to make and references therein) has led to a successful explanation of is that central cores in massive ellipticals are possible tracers of the large-scale structure in the galaxy distribution on scales violent AGN activity, and provide an alternative to binary black − 0.02 ∼< k ∼< 0.15 h Mpc 1. The CDM power spectrum on these hole scouring. If AGN are powerful sources of negative feedback scales derived from large redshift surveys such as, for instance, that cleans out the gas supply to avoid forming excessively lumi- the Anglo-Australian 2-degree Field Galaxy Redshift Survey nous and blue ellipticals, our model is a plausible and even in- (2dFGRS), is also consistent with the Lyman-α forest data in evitable precursor. These cores are seen in the stellar component, the redshift range 2 < z < 4 (Croft et al. 2002). and are likely present in the underlying (although subdominant) In spite of these impressive successes, there are still discrep- dark matter distribution. ancies between simulations and observations on scales ∼<1Mpc. Navarro et al. (1996, 1997) have shown that the spherically- We may mention the large number of sub-L∗ subhalos present averaged density profiles of simulated halos can be fitted by a in simulations but not observed (Kauffmann et al. 1993; Moore simple analytical function, depending on a characteristic den- ρ et al. 1999a; Klypin et al. 1999; but see Belokurov et al. 2006, for sity ∗ and a characteristic radius rs: ρ∗ an observational update), and the excess of massive early-type ρ r = · dm( ) 2 (1) galaxies undergoing “top-down” assembly with high inferred r/rs(1 + r/rs) specific star formation rates relative to predictions of the hier- This profile (dubbed the NFW-profile) is steeper than that of archical scenario (Glazebrook et al. 2004; Cimatti et al. 2006). an isothermal sphere at large radii and shallower close to the Here we examine the sharp central density cusp predicted by center. Steeper profiles in the central regions (α ∼−1.5) have simulations in dark matter halos and not seen in the rotation been found in high resolution simulations (Moore et al. 1999b; curves of low surface brightness galaxies (de Blok et al. 2001) Ghigna et al. 2000; Fukushige & Makino 2001). or in bright spiral galaxies (Palunas & Williams 2000; Salucci & While the “universality” of density profiles of dark halos is Burkert 2000; Gentile 2004). Little attention has been given to still a matter of debate, possibly depending on the merger history the corresponding situation in massive early-type galaxies, the (Klypin et al. 2001; Ricotti 2003; Boylan-Kolchin & Ma 2004) focus of the present analysis. or on the initial conditions (Ascasibar et al. 2004), it could nev- Our model is presented as a toy model to justify an in depth ertheless be an important key to understanding the mechanism(s) study. It is in the same spirit as that developed by Mashchenko by which these systems relax and attain equilibrium. In a colli- et al. (2006) to account for cores in dwarf galaxies. Indeed it has sionless self-gravitating system, collective mechanisms such as Article published by EDP Sciences and available at http://www.aanda.org or http://dx.doi.org/10.1051/0004-6361:20077956 124 S. Peirani et al.: Active galactic nuclei and massive galaxy cores violent relaxation and/or phase mixing certainly play a major 1e+18 role in the relaxation of halos, but density profiles may also be ff a ected by gravitational scattering of dark matter particles in 1e+17 substructures present inside halos (Ma & Bertschinger 2004a). In fact, recent numerical experiments indicate that this diffusion ) process may alter significantly the inner density profile of ha- -3 1e+16 los, producing a flattening of the original profile within a few Mpc dynamical time scales (Ma & Boylan-Kolchin 2004b). The most sun (M 1e+15 detailed study (Ricotti 2003) of N-body simulations at different ρ scales concluded that galaxies have shallower density profiles than clusters. 1e+14 Central cusps seen in simulations could also be understood as a consequence of the inflow of low-entropy material and 1e+13 therefore contain information on the relic entropy of dark mat- 0.1 1 ter particles. In fact, high resolution simulations of galaxy-sized r/rs CDM halos indicate an increase of the coarse-grained phase- Fig. 1. DM density profile of our unperturbed model. The dots are es- space density Q (defined as the ratio between the density and −1 the cube of 1-D velocity dispersion in a given volume, e.g., timates of the density at 5 h Gyr while the solid line is the expected = ρ/σ3 Hernquist profile whose relevant parameters are discussed in the text. Q ) towards the center (Taylor & Navarro 2001). Similar For comparison, the “equivalent” NFW-profile is also shown (dashed results were obtained by Rasia et al. (2003), who also obtained line). a power-law variation, e.g., Q ∝ r−β for cluster-size halos, with β quite close to the value found by Taylor & Navarro (2001), namely, β ≈ 1.87. AGN outburst can induce a bulk motion to the gas core by en- Several solutions have been proposed to explain such dis- ergy transfer. We then explore the efficiency of this mechanism crepancies between observations and numerical simulations. For of gas core bulk motions for flattening the central DM and stellar example, the dark matter (DM) can be “heated” by the baryons cusp. This paper is organized as follows: in Sect. 2, we describe by dynamical friction due to self-gravitating gas clouds orbit- our toy model, we present the results in Sect. 3, and in Sect. 4, ing near the center of the galaxy (El-Zant et al. 2001, 2004), our main conclusions are summarized. by the evolution of a stellar bar (Weinberg & Katz 2002; Holley-Bockelmann et al. 2005; Sellwood 2006), by the radia- tion recoil by a black hole (Merritt et al. 2004) or by random bulk 2. Toy model gas motions driven by supernovae feedback, recently suggested We first develop a toy model which consists of building an by Mashchenko et al. (2006). Other mechanisms have been pro- N-body realization of an isolated, equilibrium model galaxy. A posed such as the the transfer of angular momentum from bary- direct method uses the distribution function (DF), which pro- onic to dark matter (Tonini et al. 2006) or the expulsion of a vides the relative probability of a star to have a certain posi- large fraction of the gas due to feedback activities, causing the tion and velocity, and then use a Monte-Carlo sampling of the dark matter to expand (Gnedin & Zhao 2002). DF to generate the N-body realization. Thus, assuming spheri- Most of the previous studies consider the scales of dwarf cal symmetry, we generate a spherical equilibrium DM halo with galaxies or those of galaxy clusters in order to interpret the ob- a Hernquist density profile (Hernquist 1990): served DM profiles in these objects which are thought to be dark matter dominated in their inner regions. In the present paper, M a ρ (r) = dm (2) we focus our attention on the scale of a typical giant elliptical dm 2π r(r + a)3 galaxy, and we investigate the effects of a central AGN in its core. Similar but more extreme feedback ideas have been pro- where Mdm is the total mass of dark matter and a the scale 13 −1 posed for disk galaxies such as the Milky Way, where massive radius.
Details
-
File Typepdf
-
Upload Time-
-
Content LanguagesEnglish
-
Upload UserAnonymous/Not logged-in
-
File Pages7 Page
-
File Size-