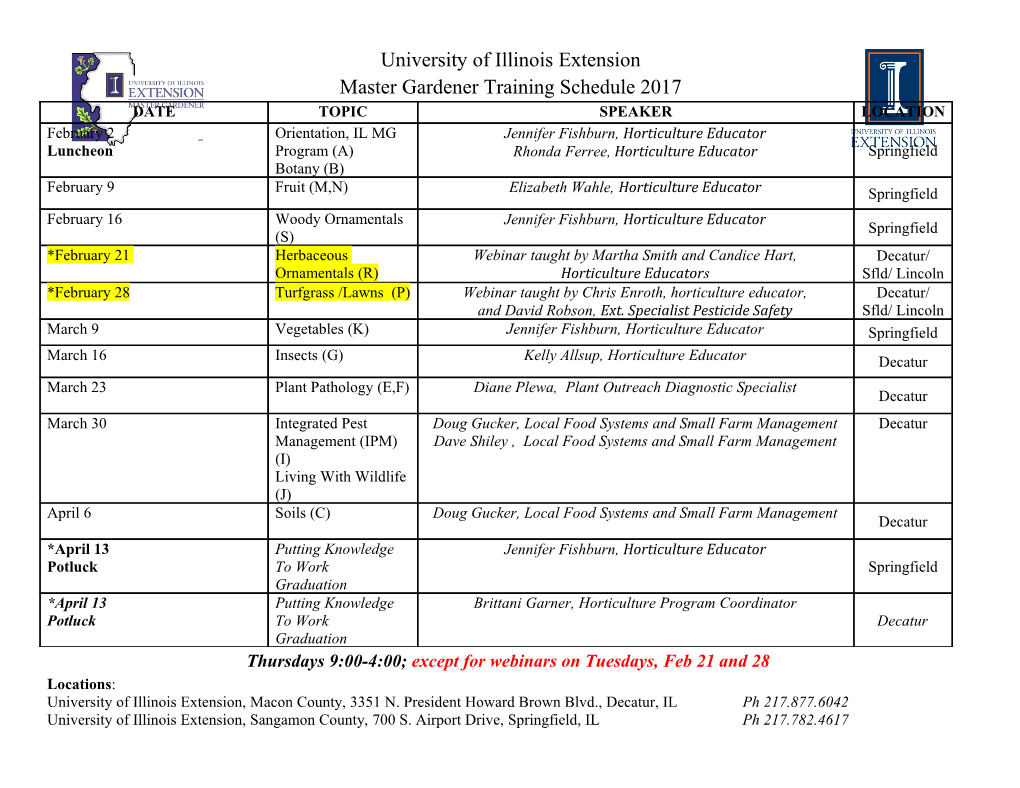
pp Collider Detectors • Aims of Collider Detectors • Special challenges at p-p(bar) colliders • The ATLAS Detector at LHC – emphasis on vertex (silicon) detectors 1 Aims of a Collider Detector Measure: – energies – directions – identities ...of the products of hard interactions as precisely as possible. 2 Particle Signatures • Stable particles (photons, electrons, muons, pions, protons, neutrons) are identified by their signatures in the detector • Unstable particles are reconstructed from their decay products, e.g. – quarks, gluons ® ‘jets’ – t ®nt + e nt or m nt or p(s) 3 Dealing with pile-up Detectors at p-p(bar) colliders must be: • very granular (to separate tracks) • very fast (to minimise # of bunch crossings per event) • radiation-hard 4 QCD Background At p-p(bar) colliders, sjets>> sinteresting, so there is huge ‘QCD background’. • Therefore, very difficult to identify interesting particles by their decays into jets. • Unfortunately BR(X ® leptons and/or photons) is usually small. Þ Must have good lepton, g identification 5 Structure of a Typical Collider Detector 6 The ATLAS Detector at LHC 7 Tracking Detectors • An Inner Tracking Detector measures the parameters of charged particles: – sign of the charge – momentum – initial direction – point of origin (vertex) • These must be measured with minimal perturbation Þ trackers must be low-mass 8 How a Tracker Does its Job • As charged particles pass through a tracking detector, they deposit energy (usually by ionisation) which can be detected. – Online: • record positions in space of the energy deposits in discrete detector elements (see next slides...) – Offline: • determine which points came from same track • fit a helix (trackers are usually immersed in a magnetic field) to these points – direction of curvature gives sign of charge – radius of curvature gives momentum • determine where tracks cross to get – points of origin – initial directions 9 The ATLAS Inner Tracker 3 sub-systems: – Silicon pixel tracker • 3 very precise measurements (‘space points’) per track • close to interaction point (R @ 5, 9, 12 cm) • Þ good vertex reconstruction – Silicon strip tracker (SCT) • back-to-back modules with relative rotation (‘stereo’) Þ3D space points • 4 precise measurements per track: 30cm < R < 52 cm – Transition Radiation Tracker (Straw Tubes) • up to 40 2D measurements per track for 56cm < R < 107cm • electron identification by Transition Radiation 10 The ATLAS Inner Tracker 11 TRT Straws - an Example of a Gaseous Tracking Detector • Passage of charged particle ionises the gas in the straw • ionisation electrons avalanche to anode wire • point of passage obtained from knowing drift time to wire 12 Silicon Detectors • A Silicon detector is analogous to a gaseous detector: – gaseous detector: electrons freed from a gas are collected on a wire – silicon: electrons freed from a crystal lattice are collected on a micro-etched metal strip. • Advantages: – Much smaller scale Þ much higher precision – charge carriers very mobile Þ fast signal collection • Disadvantages: – no charge multiplication so need good amplifiers – performance degrades with radiation damage 13 How does a Silicon Detector Work? • Energy levels in atoms become energy BANDS in crystals: thermal energy allows electrons to jump from the valence band into the conduction band • many free charge carriers :~108 in 1 cm2 of 300m-thick silicon …but a track only produces 32,000 electron-hole pairs - too small comparatively to be noticed • dope one slab of silicon with donor atoms, another with acceptor atoms. Put them together - a depletion region is formed where there are no free charges. • Bias (apply a voltage across) this ‘sandwich’ and depletion region widens; bias it enough ® full depletion. 14 How Silicon Detectors Work, cont’d • Now when a track goes through the silicon: – the 32,000 ionisation electrons are noticeable; – they drift along the internal electric field to one face of the silicon. • Put metal squares (pixels) or strips on that face to collect the ionisation electrons. – Record which pixel or strip is hit. – If using strips, must have two layers with crossed strips to get a 3D space point. 15 Cross-Section of a Silicon Detector P-type implantation Metallised pixels or strips Amplifiers 300m _ +_ n-type + Silicon + _ V Ionisation bias electrons and holes Minimum Ionising Particle 16 ATLAS Barrel SCT x 394 = 17 ATLAS Forward SCT Disks ® 18 The Atlas SCT Detector 4 barrels + 9 disks at each end: 19 Electromagnetic Calorimeters • When passing through matter, particles experience nuclear electric fields: – charged particles emit brem- strahlung radiation. • Energy loss a 1/m2, so electrons lose more energy than any other particles. – photons pair-produce (g ® e+e-). Þelectromagnetic shower • If material is thick enough, all incident energy will be deposited in the material. 20 Hadronic Calorimeters • Hadrons are affected by the residual strong forces surrounding nuclei – interaction with a nucleus leads to production of more hadrons... – ...which in turn collide with other nuclei… – This hadronic shower develops until all incident energy is absorbed. 21 Calorimeters, cont’d • EM-CAL: choose the right thickness of a high- Z material to stop electrons and photons • H-CAL: choose the right thickness of a high-A material to stop hadrons • Deposited energy is measured – entirely if absorber is active – by sampling if absorber is inactive • Shower Shapes (width of shower, depth at maximum width, ...) help distinguish e.g. electrons from hadrons. 22 ATLAS’ Calorimeters • EM calorimeter is lead/liquid Argon • Hadronic Calorimeter: – Barrel: iron/scintillator tiles – End-caps: copper/liquid Argon – Far Forward: Tungsten/liquid Argon 23 Muon Detectors • Muons are the most penetrating of the detectable particles: – heavier than electrons so don’t lose much energy in electromagnetic calorimeter – unaffected by the strong force so they aren’t absorbed in hadronic calorimeter • Muons are charged. • So muon detectors are tracking detectors located outside all other subdetectors: – All other particles are stopped by rest of detector Þ anything depositing energy in a muon detector is probably … a muon. 24 ATLAS’ muon detection system • Toroidal magnetic field is everywhere ~orthogonal to muon trajectory Þ good measurement of muon momentum • Four types of gaseous tracking chambers: – two types for tracking • Monitored Drift Tubes • Cathode Strip Chambers – two types for triggering • Resistive Plate Chambers • Thin Gap Chambers 25 What about neutrinos?? • Neutrinos almost never interact with matter. • In a perfectly hermetic detector, missing energy and momentum would signal and characterise neutrinos. • In a real detector, energy escapes (especially down the beampipe) but can measure missing transverse energy (E^) Þ E^n. 26 ATLAS Trigger / DAQ • LHC: 109 interactions per second – most are not interesting – must choose and record those that are • Event Storage Rate ~ 100 Hz Þ Major Challenge! 27 ATLAS 3-level Trigger • Level 1: fast simple algorithms in muon detector and calorimeters look for high-p^ leptons and jets. – Decision in ~2 ms. – While waiting for LVL1 decision other detectors’ data is held in pipelines – If LVL1 Yes: pipelines read out • Level 2: Interesting regions examined more carefully – slower: about 10 ms per event – LVL2-yes events passed to event filter • Event Filter: – full event is collected – some event reconstruction is performed – decision takes a few seconds per event – accepted events are stored in database 28 Further Study… See videos of J.Virdee’s lectures on particle detectors to CERN summer students: http://webcast.cern.ch/Projects/ WebLectureArchive/ssl/2000/ 0711/virdee1 Many other webcast lectures available from parent site: …WebLectureArchive/ 29.
Details
-
File Typepdf
-
Upload Time-
-
Content LanguagesEnglish
-
Upload UserAnonymous/Not logged-in
-
File Pages29 Page
-
File Size-