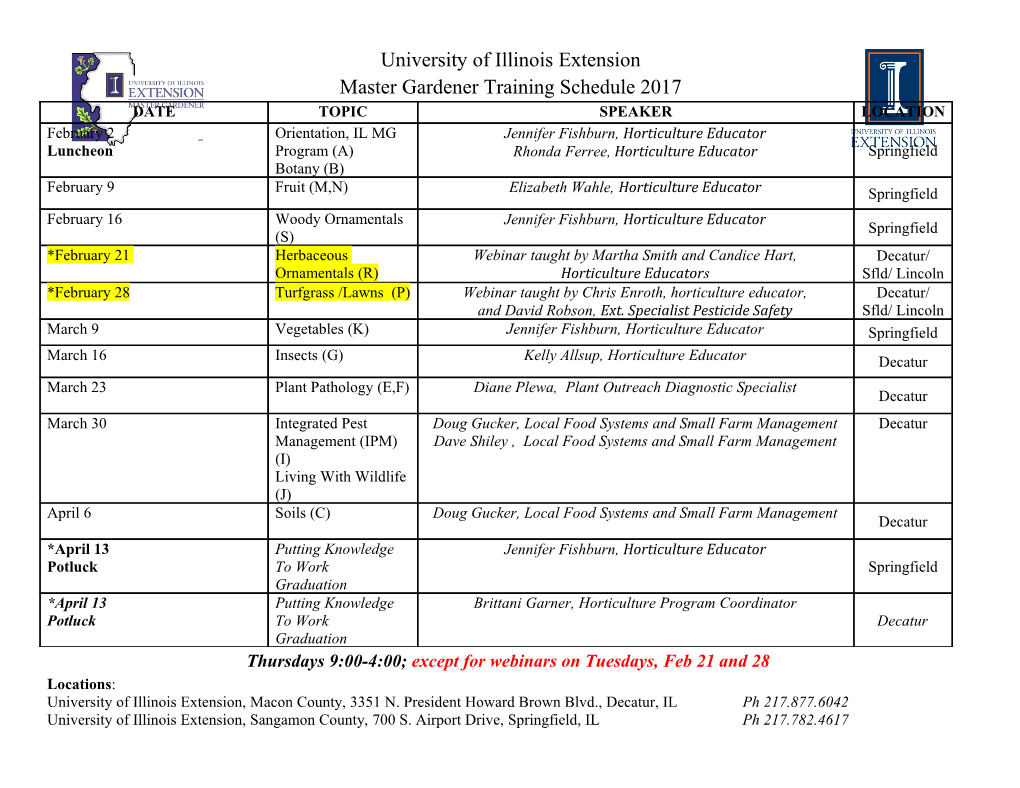
ATMS 321: Chapter 8: Past Climate Paleoclimate. Stable Isotopes: Many atoms come with different numbers of neutrons, which gives them the same chemical properties, but different masses. These are called isotopes of the elements. Examples are Oxygen, which has three important stable isotopes O16, O17 and O18. Carbon has C12 and C13. Nitrogen also has stable isotopes N14 and N15. Hydrogen comes in H and D, where D is deuterium, which is hydrogen with an extra neutron, or heavy hydrogen. Oxygen, Carbon, Nitrogen and Hydrogen are important because they are important elements for molecules that move around in the climate system, either through physiochemical processes or through biological processes. Molecules with different isotopic elements within them are called isotopologues. Important molecules that can be isotopologues are water, molecular oxygen, carbon dioxide, and many biological compounds. Lets start simply with water, H2O. Water could contain two isotopes of H and three of O. The isotopic composition of water can be measured with a mass spectrometer. Suppose you drill a core into the ice in Greenland or Antarctica, or into a mountain glacier. The time that you sample will depend on the depth of the core and the rate at which ice is accumulated. Obviously you need to be in a place where the ice accumulate year upon year, and it is better if it does not melt during part of the year, as that would cause water to percolate from the top to the bottom and confuse the time signature of the isotope signature in the ice core. We would like to be able to relate depth in the core to time in the past. Why would the isotopic concentration of the ice in the core vary over time? The answer is mass fractionation. A molecule of, say, water vapor containing a heavy isotope of Oxygen or Hydrogen will have more mass than a molecule consisting of the (more common) lighter isotopes. In Brownian Motion the heavier isotopologues of water vapor will be moving a bit slower than the lighter ones. This makes it slightly more likely that the heavy molecules will condense into liquid water as the temperature decreases to induce condensation. It also makes it slightly less likely that heavy isotopologues of liquid water will evaporate, leaping from the liquid to the vapor form. This leads us to two hypotheses: First, the lighter isotopes are more likely to evaporate. Thus the water vapor in the atmosphere is likely to have a lighter isotopic concentration than the water in the ocean. As water is removed form the ocean and deposited on land in ice sheets, then the ocean should become enriched in the heavier isotopes, as these are more likely to be left behind when ocean water is evaporated. So rather than ice sheets, lets consider first the example of the isotopes in the sediment at the bottom of the ocean. This sediment is composed of many things, but much of it is organic matter or shells from organisms that lived in the ocean, died and their carcasses or shells sank to the bottom to form sediment. A big component of this bottom sediment is calcium carbonate (CaCO3), or limestone, the shells of tiny organisms that form the base of the food pyramid in the ocean. As you can see from the formula, CaCO3 contains a lot of oxygen – three molecules of oxygen, one of calcium and one of carbon. Below is a plot of the Oxygen-18 in deep sea sediment cores. It is an indicator of global volume of ice on land. Ocean Sediment Cores Because water with lighter O16 isotope of oxygen is more likely to be evaporated, when I sheets build up on land, the ocean becomes enriched in the heavier isotope O18 in and this is recorded in the shells and biomass of organisms in ocean sediment. The mixing ratio of O18 is measured as parts per thousand deviation from the value of Standard Mean Ocean Water (SMOW) 18O / 16O " 18O / 16O 18 ( )sample ( )SMOW ! O = # 1000 18O / 16O ( )SMOW http://earthobservatory.nasa.gov/Features/Paleoclimatology_SedimentCores/ Here’s a much longer record from ocean sediment cores from Lisiecki and Raymo, 2005 PaleoOceanography Ice Cores Isotopes in Ice Cores As water vapor moves from the subtropics, where most of it is evaporated, to colder latitudes to ice caps, the heavier isotopologues of water vapor condense more readily that the lighter isotopologues. Thus the water that falls on ice sheets is even lighter than the water that is evaporated from the ocean. As the temperature above the ice sheets varies with the local climate, the isotopic signature of the water will also change, such that when the climate is colder over and near the ice sheet, the water will be lighter, and vice versa. Ice cores have been drilled an analyzed over a wide range of locations, from ice cores over Greenland in which there are annual layers, but which go back only a few thousand years, to ice sheets over Antarctica that go back up to 800,000 years. Bubbles in Ice Cores Bubbles in ice cores contain atmospheric air from the time when the bubbles were sealed in the ice. This gives a record of the composition of air deep into the past. Dust and Chemicals in Ice Cores Volcanic dust, sea salt, black carbon, desert dust and other substances borne on the air are laid down on glaciers and become part of the record as snow accumulates on them. http://earthobservatory.nasa.gov/Features/Paleoclimatology_IceCores/ Luthi et al, Nature, 453, 379 Above shows a frequency spectrum of the variance of the 2.5Myr record below. Peaks at 23, 41 and 96 thousand years correspond to peaks in summer insolation from Earths orbital parameter variations. Temperature inferred from Deuterium, a smoothed temperature record from Vostok ice core, a marine O18 record indicating global ice volume and the carbon dioxide record from the Vostok core. Stable isotopes in ice cores and temperature. An example: You have a can of BBs (small spheres of metal) in a can on your bike. The can has a hole in it. Some of the BB’s are made of copper and some are made of stainless steel. Although the BBs are the same size, the stainless steel ones are slicker and denser and tend to fall out of the hole a little more easily than the copper BBs. The road is rough and the can gets shaken so that the steel and copper BBs are always well mixed up in the can. You fill the can with BBs that are half copper and half stainless steel, put the can on the back of your bike and start to ride toward Grandma’s house. The stainless steel BB’s are more likely to fall out as the copper ones. How does the proportion of steel to copper BBs change as you ride toward Grandma’s house. Let’s suppose that the number of BB’s lost per unit time depends on the number of BB’s still in the can. Then we could write that, dN dN s = ! p N c = ! p N dt s s dt c c Where Ns is the number of steel BBs and Nc is the number of copper ones. If we start out with Nso steel balls and Nco copper balls we can solve for the number of balls of each type as a function of time. Ns = Nso exp(! pst) Nc = Nco exp(! pct) Then the ratio of steel balls to copper balls is, S Nso = exp((pc ! ps )t) C Nco If the rate of loss of steel balls is greater than the loss rate of copper balls (ps > pt), then this ratio, S/C, will decrease with time. If you replace steel balls with heavy isotopes and copper balls with light isotopes and you replace time with temperature, then you have some idea why the ratio of heavy isotopes to light ones decreases as cooling causes preferential condensation of heavier isotopes, so that ice formed at colder temperatures is isotopically lighter than that formed at higher temperatures. Surface of Earth at time of last glacial maximum. Black area contours are ice sheet depth in meters. This diagram shows Temperature, non-sea-salt sulfate and non-sea-salt Calcium in 140,000-year record from Vostok Ice Core. Note that ice ages are dusty on Antarctica. This is consistent with land evidence for a drier, windier time then. .
Details
-
File Typepdf
-
Upload Time-
-
Content LanguagesEnglish
-
Upload UserAnonymous/Not logged-in
-
File Pages14 Page
-
File Size-