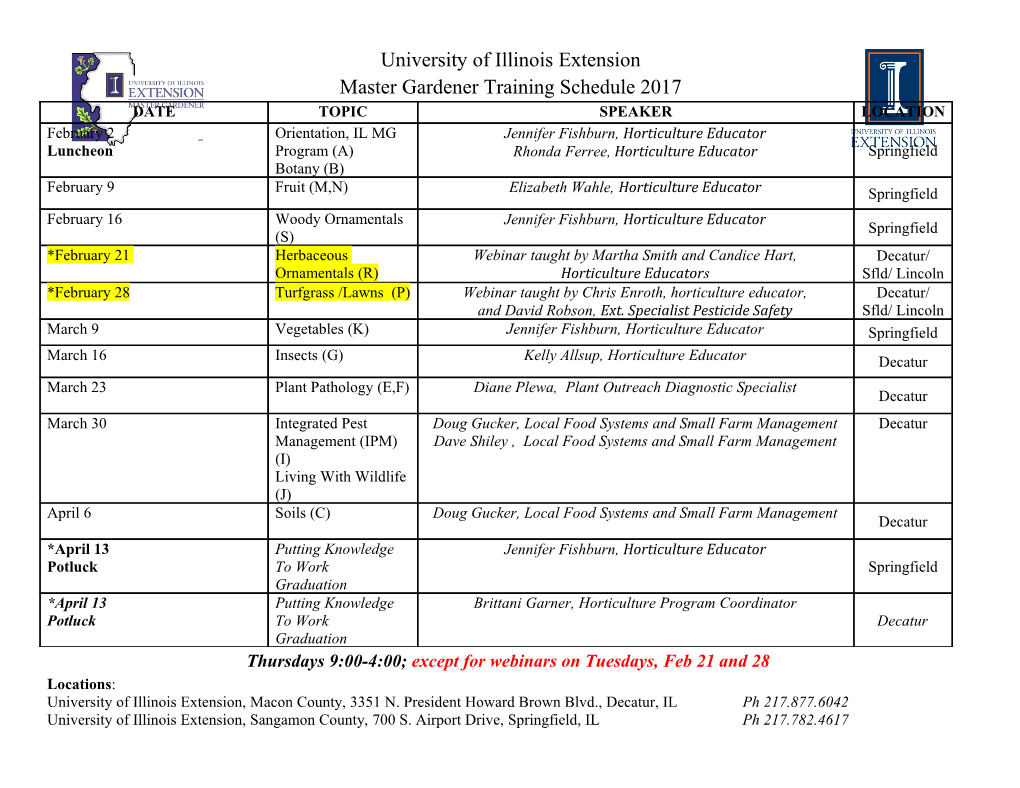
ARTICLE Received 4 Nov 2012 | Accepted 3 May 2013 | Published 10 Jun 2013 DOI: 10.1038/ncomms2981 Role of biogenic silica in the removal of iron from the Antarctic seas Ellery D. Ingall1,*, Julia M. Diaz1,*,w, Amelia F. Longo1, Michelle Oakes1,w, Lydia Finney2, Stefan Vogt2, Barry Lai2, Patricia L. Yager3, Benjamin S. Twining4 & Jay A. Brandes5 Iron has a key role in controlling biological production in the Southern Ocean, yet the mechanisms regulating iron availability in this and other ocean regions are not completely understood. Here, based on analysis of living phytoplankton in the coastal seas of West Antarctica, we present a new pathway for iron removal from marine systems involving structural incorporation of reduced, organic iron into biogenic silica. Export of iron incorpo- rated into biogenic silica may represent a substantial unaccounted loss of iron from marine systems. For example, in the Ross Sea, burial of iron incorporated into biogenic silica is conservatively estimated as 11 mmol m À 2 per year, which is in the same range as the major bioavailable iron inputs to this region. As a major sink of bioavailable iron, incorporation of iron into biogenic silica may shift microbial population structure towards taxa with relatively lower iron requirements, and may reduce ecosystem productivity and associated carbon sequestration. 1 School of Earth and Atmospheric Sciences, Georgia Institute of Technology, 311 Ferst Drive, Atlanta, Georgia 30332-0340, USA. 2 Advanced Photon Source, Argonne National Laboratory, 9700 South Cass Avenue, Argonne, Illinois 60439, USA. 3 Department of Marine Sciences, University of Georgia, 220 Marine Sciences Building, Athens, Georgia 30602, USA. 4 Bigelow Laboratory for Ocean Sciences, 60 Bigelow Drive, East Boothbay, Maine 04544, USA. 5 Skidaway Institute of Oceanography, 10 Ocean Science Circle, Savannah, Georgia 31411, USA. * These authors contributed equally to this work. w Present address: Biology Department, Woods Hole Oceanographic Institution, 226 Woods Hole Road, Woods Hole, Massachusetts 02543, USA (J.M.D.); Environmental Protection Agency, National Center of Environmental Assessment, Research Triangle Park, North Carolina 27711, USA (M.O.). Correspondence and requests for materials should be addressed to E.D.I. (email: [email protected]). NATURE COMMUNICATIONS | 4:1981 | DOI: 10.1038/ncomms2981 | www.nature.com/naturecommunications 1 & 2013 Macmillan Publishers Limited. All rights reserved. ARTICLE NATURE COMMUNICATIONS | DOI: 10.1038/ncomms2981 iliceous phytoplankton are abundant and productive components of marine ecosystems, with diatoms Si Fe Saccounting for B43% of annual marine carbon fixation worldwide1. Sedimentation of the dense silica produced by these microorganisms has a critical role in the transport of carbon to the deep ocean2 and may also enhance the export of phosphorus and nitrogen below the mixed layer3,4. Previous observations of iron in siliceous frustules of cultured diatoms5 suggests that sedimentation of biogenic silica may also be a quantitatively significant mechanism for the removal of bioavailable iron. Yet, 205 28.9 this potential mechanism has remained largely unexplored in natural settings. Diatoms often dominate phytoplankton communities in seasonally ice-covered Antarctic coastal seas, such as the Ross Si Fe Sea6. Primary production and microbial community composition in this region are variably limited by a number of physical conditions and nutrients, including iron7–9, which in turn affects biological carbon dioxide uptake and therefore climate6. Although these waters are the most productive regions of the Southern Ocean10 and major sites of atmospheric carbon dioxide uptake11, the mechanisms governing the cycling of the limiting 8 nutrient iron are not well understood in these and other ocean 110 0.225 areas. On the basis of this gap in knowledge, combined with renewed interest in iron fertilization as an approach to the current 12 climate crisis , an improved understanding of iron cycling in Si Fe marine systems is critical. Building on studies in which total iron quotas of single cells were quantified13, we present both the content and speciation of iron contained in one biological pool relatively less subject to remineralization: iron incorporated into biogenic silica. We show this pool represents a significant iron removal mechanism from Ross Sea surface waters. Results Chemical treatments. Diatoms of the genus Fragilariopsis 74.5 0.507 dominated the community of siliceous plankton sampled along a transect of the Western Antarctic coastal ocean, followed by Figure 1 | XRF micrographs. Maps of iron (Fe) and silicon (Si) content in silicoflagellates. Subsets of these specimens were chemically siliceous specimens, including Corethron spp. (a), Fragilariopsis spp. (b) and treated with trace metal grade reagents to isolate the pool of iron silicoflagellates (c) revealed two morphologically distinct iron pools incorporated into silica. These treatments included washes with associated with biogenic silica: iron colocalizing with silica (stars) and 6 N hydrochloric acid, which removed organic coatings and micron-sized iron hotspots (arrows). Stars and arrows indicate regions that loosely associated iron, or 30% hydrogen peroxide, which targeted were further investigated with iron XANES. The number in the lower left organic iron material on the silica surface14. Sixty-six individual corner of each image is the maximum elemental content (mgcmÀ 2) specimens (17 untreated, 26 peroxide washed and 23 acid represented by white (elemental content scales linearly, with black as zero). washed) were analysed using X-ray fluorescence (XRF) The heights of images in rows a, b and c are 66, 27 and 26 mm, respectively. microscopy and X-ray absorption near edge structure (XANES) All images were collected from untreated samples (that is, no peroxide or spectroscopy. acid treatment). Iron morphology. XRF micrographs of single specimens revealed another strong indicator of iron oxidation state15, revealed a two morphologically distinct forms of iron: (1) iron that mirrored continuum of oxidation states, with incorporated iron being more silicon distributions and (2) occasional micron-sized regions of reduced and iron hotspots being more oxidized (Fig. 4). Third, high iron content (termed hotspots) (Fig. 1). The per-pixel iron based on comparison of our specimens to XANES spectra of content of the former correlated strongly with per-pixel silicon standard materials using linear combination fitting, incorporated content in each specimen, confirming structural incorporation of iron was best accounted for by B50 (±14)% each of iron (II) iron into biogenic silica (Fig. 2). This pattern was much unlike the acetate and the iron (III) oxide goethite (Fig. 3), suggesting the distribution of other trace metals, such as manganese, which presence of a significant fraction of reduced organic iron. On the appeared to be surface associated (Fig. 2). other hand, iron hotspots were best fitted by minerals containing oxidized iron (that is, B66 (±24)% haematite and 34 (±24)% Iron speciation. Several lines of evidence generated from the goethite on average) (Fig. 3). Finally, the reduced oxidation state XANES analysis demonstrate that iron incorporated into biogenic of iron colocalizing with silicon supports the conclusion that iron silica is reduced and likely present in organic form. First, the is structurally incorporated and is, therefore, a natural component average XANES spectrum of iron incorporated into silica exhib- of biogenic silica, as any reduced iron unprotected from the ited a K-edge position that was substantially lower than that of ambient surface seawater would rapidly oxidize. The range of iron the average iron hotspot by 2.8±0.3 eV (Fig. 3). This lower oxidation states observed in structurally incorporated iron might K-edge is consistent with a reduced oxidation state of iron in the reflect gradual oxidation of iron over time, or a naturally mixed biogenic silica. Second, the position of the pre-edge centroid, redox composition. 2 NATURE COMMUNICATIONS | 4:1981 | DOI: 10.1038/ncomms2981 | www.nature.com/naturecommunications & 2013 Macmillan Publishers Limited. All rights reserved. NATURE COMMUNICATIONS | DOI: 10.1038/ncomms2981 ARTICLE 3×10–6 3×10–6 3×10–6 ) ) ) –2 –2 –2 Fe (mmol cm Fe (mmol cm Fe (mmol cm R 2=0.89 R 2=0.68 R 2=0.92 0 0 0 0 4.5×10–6 0 4.5×10–6 0 4.5×10–6 Si (mol cm–2) Si (mol cm–2) Si (mol cm–2) 8×10–7 8×10–7 8×10–7 ) ) ) –2 –2 –2 Mn (mmol cm Mn (mmol cm R 2=0.25 R 2=0.08 Mn (mmol cm R 2=0.45 0 0 0 0 4.5×10–6 0 4.5×10–6 0 4.5×10–6 Si (mol cm–2) Si (mol cm–2) Si (mol cm–2) Figure 2 | Scatter plots of elemental content. Typical data collected from three individual specimens of Fragilariopsis spp. (such as those in Fig. 1b) are displayed as scatter plots of per-pixel elemental content. In all specimens, the pool of iron (Fe) that colocalized with silicon (Si), that is, excluding hotspots, correlated positively with silicon content (a–c). This pattern is consistent with structural incorporation of iron, as thicker areas of silicon correspond to a proportionally higher content of iron. In contrast, manganese (Mn) is typically associated with particles, such as biogenic silica, as a surface coating. Consistent with this surface distribution, Mn content did not exhibit a relationship with Si content (d–f). Scatter plots in each column were generated from the same single specimen. All plots were generated from untreated samples (that is, no peroxide or acid treatment). ab1.2 1.2 c1.2 Incorporated iron 1 1 1 Hotspot iron 0.8 0.8 0.8 1 0.6 0.6 0.6 1 0.4 0.4 0.4 Relative intensity Relative intensity Relative intensity 0.2 2 0.2 2 0.2 0 0 0 7.08 7.10 7.12 7.14 7.16 7.18 7.08 7.10 7.12 7.14 7.16 7.18 7.115 7.120 7.125 7.130 7.135 Energy (keV) Energy (keV) Energy (keV) Figure 3 | Iron XANES spectra.
Details
-
File Typepdf
-
Upload Time-
-
Content LanguagesEnglish
-
Upload UserAnonymous/Not logged-in
-
File Pages6 Page
-
File Size-