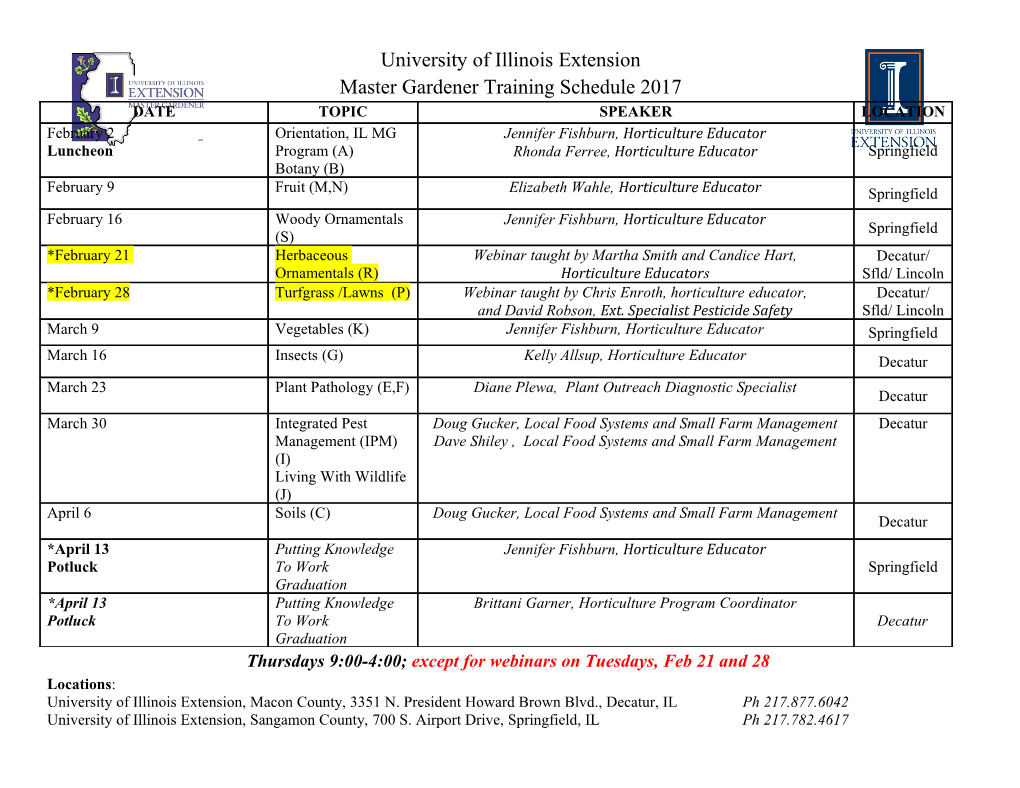
X-10 REACTOR FORENSIC ANALYSIS AND EVALUATION USING A SUITE OF NEUTRON TRANSPORT CODES A Thesis Presented to The Academic Faculty by Evan Maxwell Redd In Partial Fulfillment of the Requirements for the Degree Master of Science in Nuclear Engineering in the George W. Woodruff School of Mechanical Engineering Georgia Institute of Technology August 2015 COPYRIGHT © 2015 EVAN MAXWELL REDD X-10 REACTOR FORENSIC ANALYSIS AND EVALUATION USING A SUITE OF NEUTRON TRANSPORT CODES Approved by: Dr. Anna Erickson, Advisor Dr. Ce Yi School of Mechanical Engineering School of Mechanical Engineering Georgia Institute of Technology Georgia Institute of Technology Dr. Glenn Sjoden Air Force Technical Applications Center U.S. Air Force Dr. Kevin Manalo High Performance Computing Alabama School System HPC Date Approved: March 25, 2015 This thesis work is dedicated to my family. To my father, Alan, who taught me to never give up and to never stop learning. My late mother, Linda, who instilled the importance of happiness and selflessness. My sister, Lindsay, who showed me strong resolve and persistence in achieving happiness. Last but not least, my beautiful wife Michelle, who unconditionally supported and encouraged me throughout my academic career and life. ACKNOWLEDGEMENTS First, I would like to thank Dr. Glenn Sjoden for his career/research guidance and challenging reactor physics courses that imparted the skills needed for becoming a successful nuclear engineer. I would also like to thank Dr. Anna Erickson, who provided a smooth transition in advisement and was instrumental in shaping this research. Last and certainly not least, I would like to thank the former CRiTCEL team. Specifically, Dr. Kevin Manalo, Dr. Ce Yi, Dr. Chris Edgar, Mike Chin, Dr. Jessica Paul and Matt Molinar for assistance and most importantly the patience for fielding my endless questions. This material is based upon work supported by the U.S. Department of Homeland Security under Grant Award Number, 2012-DN-130-NF0001. The views and conclusions contained in this document are those of the author(s) and should not be interpreted as representing the official policies, either expressed or implied, of the U.S. Department of Homeland Security. iv TABLE OF CONTENTS Page ACKNOWLEDGEMENTS iv LIST OF TABLES vii LIST OF FIGURES viii SUMMARY xii CHAPTER 1 Introduction 1 1.1 History of X-10 2 1.1.1 X-10 Graphite Reactor Structure and Fuel Elements 3 1.2 Forensic Relevance 7 1.3 Problem Statement 10 2 Forensic Analysis Isotope/Ratios of Interest 12 2.1 Operating Neutron Spectrum and Enrichment from Plutonium Isotopics12 2.2 Relative Fuel Type Fissions in Reactor Operation 15 2.3 Xe-135/Xe-133 Ratio and Fission Spectrum Type 21 2.4 Concluding Forensic Analysis Remarks 22 3 X-10 Unit Cell Simulations and Impact on Forensic Analysis 23 3.1 Unit Cell Parameters 24 3.2 MCNP 26 3.2.1 MCNP Eigenvalue 26 3.2.2 MCNP Burnup 30 3.3 Scale 38 3.3.1 Scale Eigenvalue 38 v 3.3.2 Scale Burnup 40 3.4 PENTRAN 48 3.4.1 PENTRAN Eigenvalue 49 3.4.2 PENTRAN Burnup 56 3.5 Comparison and Impact of Unit Cell Solutions 62 3.5.1 Comparison of Eigenvalue Calculations 63 3.5.2 Comparison of Burnup/Depletion Calculations between Monte Carlo Transport Codes 65 3.5.3 Comparison of Burnup/Depletion Calculations between Monte Carlo and Deterministic Transport Codes 72 4 X-10 Eighth-Core Simulations and Impact on Forensic Analysis 78 4.1 Eighth-Core Parameters 78 4.2 Scale 81 4.2.1 Scale Eigenvalue 82 4.2.2 Scale Burnup 85 4.2.3 Scale Uncertainty 87 5 Forensic Assessment of X-10 Simulation Results 101 5.1 Outcomes Based on Assumed Operating Constraints 101 5.2 Forensic Evaluation of Burnup Results 104 5.3 Forensic Comparative Perspective of X-10 Signatures 111 6 Overall Conclusions and Recommendations 115 6.1 Computational and Forensic Conclusions 116 6.2 Future Considerations 119 REFERENCES 120 vi LIST OF TABLES Page Table 2.1: Total Fissions Isotope Indicators 19 Table 2.2: Fission Types Isotope Indicators 20 Table 3.1: MCNP Unit Cell Eigenvalue Calculation Results 27 Table 3.2: Energy Ranges of Fissions within Unit Cell 29 Table 3.3: Normalized Fluxes within Fuel 29 Table 3.4: Normalized Fluxes within Moderator 29 Table 3.5: Pu-239 and Pu-240 Mass at Discharge 37 Table 3.6: Scale Unit Cell Eigenvalue Calculation Results 39 Table 3.7: PENTRAN Unit Cell Eigenvalue Calculation Results 49 Table 3.8: PENTRAN Homogenized Unit Cell Eigenvalue Calculation Results 51 Table 3.9: Eigenvalue Calculation Comparisons between Codes 63 vii LIST OF FIGURES Page Figure 1.1: X-10 Reactor Schematic 4 Figure 1.2: Graphite Block Placement 5 Figure 2.1: Principle Neutron Capture Reactions 13 Figure 2.2: Pu-240 Capture and Pu-241 Fission Cross Sections 14 Figure 2.3: Various Total Fission Cross-Sections 16 Figure 2.4: Thermal Fission Yield Curve (U-235 and Pu-239) 17 Figure 3.1: MCNP k ∞ Values Across Burnup 32 Figure 3.2: MCNP k ∞ Behavior across 45.47 Days of Burnup 32 Figure 3.3: MCNP Calculated Xe-135 Poison Effect on k ∞ 33 Figure 3.4: MCNP Calculated Eu-154/Cs-137 Ratio across Burnup 34 Figure 3.5: MCNP Calculated Ru-106/Ba-138 Ratio Related to Pu Purity 35 Figure 3.6: MCNP Calculated Zr-94/Cs-137 Ratio across Burnup 36 Figure 3.7: MCNP Calculated Ba-138/Total Ba Related to Pu Purity 37 Figure 3.8: Scale k ∞ Values Across Burnup 41 Figure 3.9: Scale Calculated Ru-106/Ba-138 across Burnup 42 Figure 3.10: Scale Calculated Ba-138 Content across Burnup 43 Figure 3.11: Scale Calculated Ru-106 Content across Burnup 44 Figure 3.12: Scale Calculated Zr-94/Cs-137 Ratio across Burnup 45 Figure 3.13: Scale Calculated Neutron Spectrum and Enrichment Sensitive Ratios 46 Figure 3.14: Scale Calculated Neutron Spectrum and Enrichment Sensitive Ratios after 179 Days of Operation 47 Figure 3.15: Scale Calculated Xe-135/Xe-133 Mass Ratio across Burnup 48 Figure 3.16: PENTRAN Homogenized Unit Cell Depiction 51 viii Figure 3.17: PENTRAN 3D Fast Flux Plot 52 Figure 3.18: PENTRAN 3D Epithermal Flux Plot 52 Figure 3.19: PENTRAN 3D Thermal Flux Plot 53 Figure 3.20: PENTRAN Fast Flux Distribution Plot 54 Figure 3.21: PENTRAN Epithermal Flux Distribution Plot 55 Figure 3.22: PENTRAN Thermal Flux Distribution Plot 55 Figure 3.23: PENTRAN k∞ Values across Burnup 57 Figure 3.24: PENTRAN Calculated Xe-135 Behavior across Burnup 58 Figure 3.25: PENTRAN Calculated Ba-137 Behavior across Burnup 59 Figure 3.26: PENTRAN Calculated Pd-106 Behavior across Burnup 60 Figure 3.27: PENTRAN Calculated Ag-107 Behavior across Burnup 61 Figure 3.28: PENTRAN Calculated Pu-240/Pu-239 Ratio Behavior across Burnup 62 Figure 3.29: Pu-239 Mass Difference between Monte Carlo Codes 66 Figure 3.30: Fissile Pu/Total Pu Behavior between Monte Carlo Codes 67 Figure 3.31: Fissile Pu/Total Pu Behavior between Monte Carlo Codes (NRX Design) 68 Figure 3.32: Cs-137 Mass Difference between Monte Carlo Codes 69 Figure 3.33: Ru-106 Behavior between Monte Carlo Codes 70 Figure 3.34: Ba-138/Total Ba Behavior between Monte Carlo Codes 71 Figure 3.35: Pu-239 Calculated Mass between Deterministic and Monte Carlo Codes 73 Figure 3.36: Pu-241 Calculated Mass between Deterministic and Monte Carlo Codes 74 Figure 3.37: Cs-137 Calculated Mass between Deterministic and Monte Carlo Codes 75 Figure 3.38: Ce-140 Calculated Mass between Deterministic and Monte Carlo Codes 76 Figure 4.1: X-10 Reactor Fuel Loading Scheme 79 Figure 4.2: Eighth-Core Division of the X-10 Reactor 80 Figure 4.3: X-10 Reactor Channel Simulation Locations 82 ix Figure 4.4: Eighth-Core Average k-effective Plot (BOL) 83 Figure 4.5: Eighth-Core Average k-effective Plot (SS) 83 Figure 4.6: Eighth-Core Average k-effective Plot (EOL) 84 Figure 4.7: Axial Flux Distribution for Selected Channels 86 Figure 4.8: Pu-239 Axial Mass Distribution for Zone 7 Channel 87 Figure 4.9: Pu-240/Pu-239 Axial Ratio Distribution for Zone 7 Channel 88 Figure 4.10: Pu-240/Pu-239 Axial Ratio Distribution near Centerline of Zone 7 89 Figure 4.11: BOL and EOL Pu-240/Pu-239 Axial Ratio Distribution of Zone 7 90 Figure 4.12: Eighth-Core SS Neutron Fission and Absorption Spectrum 91 Figure 4.13: Eighth-Core BOL and EOL Chi Distribution 92 Figure 4.14: Eighth-Core Normalized Flux Distribution 93 Figure 4.15: Eighth-Core Model Depiction 95 Figure 4.16: Eighth-Core Total Pu Production between Monte Carlo Codes 96 Figure 4.17: Average Pu-242/Pu-238 Ratio According to Zones in Reactor 98 Figure 4.18: Decayed and SS Pu-242/Pu-238 Ratio According to Zones in Reactor 99 Figure 4.19: Decayed and SS Pu-242/Pu-240 Ratio According to Zones in Reactor 100 Figure 5.1: Pu-240/Pu-239 Unit Cell and Eighth-Core Ratio Compared 103 Figure 5.2: Pu-240/Pu-239 Ratio Comparison between Monte Carlo Codes with Nuclear Data Uncertainty 104 Figure 5.3: Pu-240/Pu-239 Ratio Comparison between Monte Carlo and Deterministic Codes with Nuclear Data Uncertainty 105 Figure 5.4: Pd-106 Mass Prediction between Monte Carlo and Deterministic Codes 107 Figure 5.5: Percent Change of Pu Isotopes across Burnup 108 Figure 5.6: Percent Change of Major Pu Ratios across Burnup 108 Figure 5.7: Pu-239 and U-235 Nubar and Percent Difference 109 Figure 5.8: Eighth-Core Pu Production with and without Carbon in Fuel 110 x Figure 5.9: PWR Pu-240/Pu-239 Ratio Compared to X-10 111 Figure 5.10: Reactor Intent Plot between PWR and X-10 113 Figure 5.11: Pu-242/Pu-238 Ratio Comparison between PWR and X-10 114 xi SUMMARY X-10, the genesis production reactor for the U.S.
Details
-
File Typepdf
-
Upload Time-
-
Content LanguagesEnglish
-
Upload UserAnonymous/Not logged-in
-
File Pages134 Page
-
File Size-