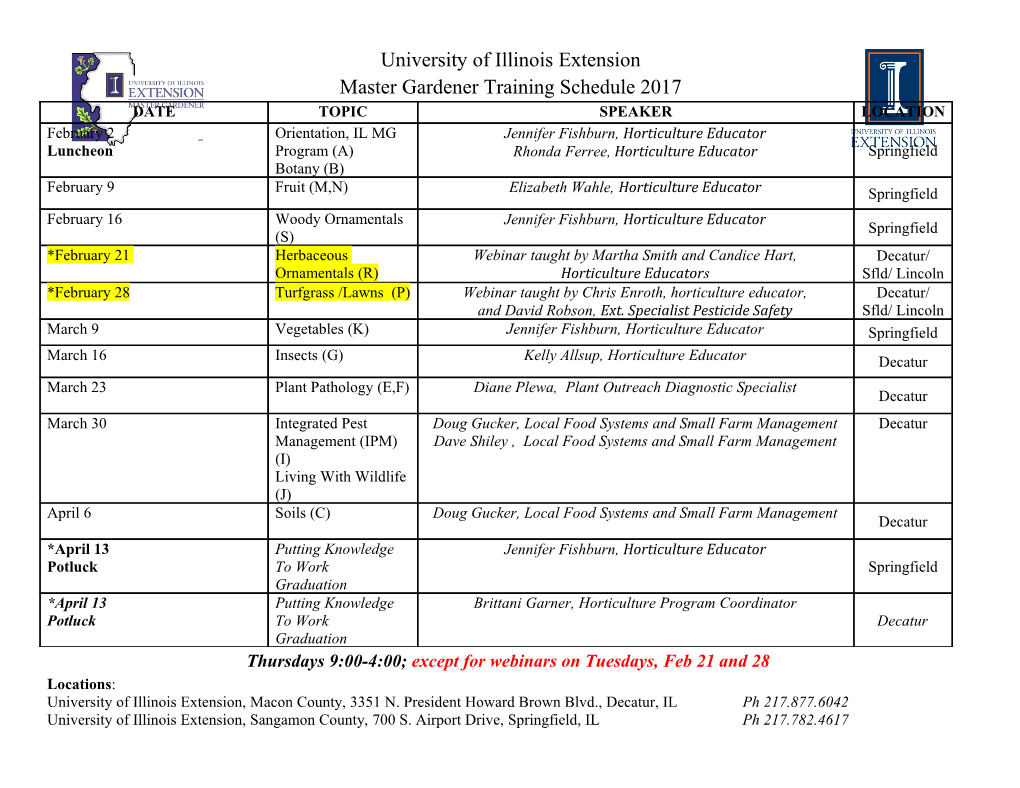
Optically triggering spatiotemporally confined GPCR PNAS PLUS activity in a cell and programming neurite initiation and extension W. K. Ajith Karunarathnea, Lopamudra Giria, Vani Kalyanaramana, and N. Gautama,b,1 Departments of aAnesthesiology and bGenetics, Washington University School of Medicine, St. Louis, MO 63110 Edited* by Peter N. Devreotes, Johns Hopkins University School of Medicine, Baltimore, MD, and approved February 12, 2013 (received for review November 29, 2012) G-protein–coupled receptor (GPCR) activity gradients evoke impor- due to the known high light sensitivity, slow deactivation (4), and tant cell behavior but there is a dearth of methods to induce such rapid bleaching (9) of rhodopsin. asymmetric signaling in a cell. Here we achieved reversible, rapidly Opsins are optimally activated at wavelengths that span the switchable patterns of spatiotemporally restricted GPCR activity in entire visual spectrum (4). We developed a strategy to identify a single cell. We recruited properties of nonrhodopsin opsins—rapid opsins with sufficient spectral selectivity, such that the wave- deactivation, distinct spectral tuning, and resistance to bleaching— length used to globally image cellular and molecular responses to activate native Gi, Gq, or Gs signaling in selected regions of a cell. using fluorescent protein reporters did not interfere with the Optical inputs were designed to spatiotemporally control levels of localized optical activation of an opsin. Using this strategy, we second messengers, IP3, phosphatidylinositol (3,4,5)-triphosphate, developed a set of optical triggers based on nonrhodopsin opsins and cAMP in a cell. Spectrally selective imaging was accomplished that are capable of selectively modulating the activity of all three to simultaneously monitor optically evoked molecular and cellular major heterotrimeric G proteins—Gi/o, Gq, and Gs—in selected response dynamics. We show that localized optical activation of an opsin-based trigger can induce neurite initiation, phosphatidy- regions of a cell. linositol (3,4,5)-triphosphate increase, and actin remodeling. Serial We then examined whether these optical triggers could be CELL BIOLOGY optical inputs to neurite tips can refashion early neuron differen- used to achieve optical control over polarized cell behavior that tiation. Methods here can be widely applied to program GPCR- is regulated by GPCR activity. Because global activation of Gi/ mediated cell behaviors. o-coupled GPCRs induces neurite outgrowth (10), we examined whether neurite growth can be spatially controlled by optically lo- optogenetics | cell polarity calizing GPCR signaling in a cell. We found that optical activation of the opsin-based signaling triggers can achieve coordinated con- -protein–coupled receptors (GPCRs) initiate most of the trol over early neuron differentiation events. Gsignaling in metazoans and regulate a wide variety of cel- Results lular responses that include differentiation, migration, secretion, Screening Opsins for Spectral Selectivity. The three human color and contraction. Asymmetric activation of GPCR signaling ac- fi tivity in a cell is thought to play a critical role in varied processes opsins, blue, green, and red have been identi ed and charac- such as cell polarization (1) and modulation of neuron function terized biochemically (11, 12). These opsins absorb maximally at ∼ ∼ (2). There is still limited information about activation of sig- 414 nm (blue), 540 nm (green), and 560 nm (red) (11, 12) naling that is restricted in space and time across a single cell. An (Fig. 1A). However, their ability to function heterologously in an impediment is the lack of methods to continuously vary signal intact cell has not been examined. Color opsins are coupled to α input to a single cell with high time resolution and precision to the G-protein subunit, G tc in the cone photoreceptor cells of α quantitate second messenger and cellular output from the same the mammalian retina (13). Because G tc is homologous to and cell. A method that provides reversible, temporal control over falls in the Gi subfamily (14), we examined whether the human GPCR activity in restricted regions of a single cell may help govern cell behavior and probe the cellular and molecular basis Significance of single-cell responses. Here we used a set of light-triggered GPCRs, human color G-protein–coupled receptors control a variety of important cell opsins, and related nonrhodopsin opsins, to achieve such confined behaviors. However, tools are not available to activate these GPCR activation in a single cell. In contrast to molecular gra- receptors in selected areas of a cell and exert control over cell dients, an optical signal provides higher spatiotemporal control behavior. Here we recruit unique properties of nonrhodopsin and it can be switched on or off or relocated almost instan- opsins to activate all the major types of G-protein signaling in taneously. We recruited the properties of color opsins to develop spatially confined regions of single cells. We show that this optical triggers that spatiotemporally confine signaling. These approach can be used to optically induce polarized cell be- opsins deactivate rapidly and demonstrate relatively low sensi- havior and refashion early neuron differentiation. This optical tivity to light (3, 4). The deactivation characteristics curtail the approach can be applied to control other cell behaviors such as diffusion of activated receptors across a cell and help localize immune cell migration and cardiomyocyte contraction. receptor activation to selected regions of a cell. Low susceptibility Author contributions: W.K.A.K. and N.G. designed research; W.K.A.K. performed re- to bleaching allows continuous reproducible activation. In addi- search; W.K.A.K. and V.K. contributed new reagents/analytic tools; W.K.A.K. and L.G. tion, nonrhodopsin opsins selectively activate different G-protein analyzed data; and W.K.A.K., L.G., and N.G. wrote the paper. types, suggesting that they can be individually used to regulate The authors declare no conflict of interest. distinct second messengers (4, 5). Rhodopsin or its chimeric *This Direct Submission article had a prearranged editor. – forms have been valuable for globally activating G proteins (6 8). 1To whom correspondence should be addressed. E-mail: [email protected]. However, we found that these receptors do not allow spatial This article contains supporting information online at www.pnas.org/lookup/suppl/doi:10. control over G-protein activation in a single cell. This is likely 1073/pnas.1220697110/-/DCSupplemental. www.pnas.org/cgi/doi/10.1073/pnas.1220697110 PNAS Early Edition | 1of10 Downloaded by guest on September 29, 2021 A B Red Green Blue Jellyfish Melanopsin Select an opsin ~560nm ~540 ~414 ~500 ~480 445 nm 488 nm Choose a wavelength 515 nm 595 nm αi/ο β γ αi/ο β γ α i /ο β γ αs β γ αq β γ Set condions for imaging Intensity (I), exposure (E), Change repeats (N) wavelength C Before After Retinal YFP Image FP-γ9 Change imaging + Green Opsin +YFP γ9 translocaon in cells condions (I, E, N) opsin 515 1.2 Red opsin bOpsin+mChγ9 expressing the opsin +CFP γ9 (without retinal) NO CFP 1.0 bOpsin+mChγ9 + (with retinal) Is γ9 0.8 translocaon YES Lowest YES 445 detectable ? I, E, and N? mCh 0.6 - 0.4 5 μW OI NO 595 445nm The opsin is spectrally selecve 0.2 under these opcal condions Normalized IM fluorescence bOpsin Red opsin Green + 0.0 mCh 595 0 3 6 9 12 20 40 60 80 100 Time (s) E Optical Imaging D Single point laser beam Designing an opcal input activation response (Unit opcal input) λ1 λ2 optical input (OI) cell Intensity 2 10 Duraon = t Time OI area (mm) X 0.87 (ms/mm) 0 1 0 5 t = + 0 1 2 mm 0 5 10 mm imaging exposure time (ms) FWHM=0.8 mm F Before After 1.0 G 0.8 9 βγ 0.6 9 fluorescence γ 0.4 9 fluorescence βγ 0.2 Internal membrane change in internal membranes Fractional GFP- 0.0 Time taken to reach max fluorescence intensity (s) 0246 445 nm Beam energy ( μW) Time (s) Fig. 1. Screening opsins for spectral selectivity and optimizing conditions for OIs and imaging. (A) Opsins used, their λ-max, and Gα-subtype specificity are shown. Individual opsins are described in the text. (B) Schematic for screening opsins for spectral selectivity. (C) Representative images of HeLa cells expressing green opsin and YFP-γ9 (green), red opsin and CFP-γ9 (blue), and bOpsin and mCh-γ9 (red). Cells were incubated with (+) or without (−) 11-cis retinal as indicated. For green opsin and red opsin, FP-γ9 distribution in the first (before) and last (after) images during image capture is shown. For bOpsin, images show mCh-γ9 distribution before and after optical activation (at 20 s after initiating image acquisition) with 445-nm, 5-μW optical inputs. Translocation of FP- βγ to intracellular membranes (IM) is plotted on the right (n = 8). bOpsin-expressing cells with retinal were activated with a single 5-μW pulse whereas cells without retinal did not show translocation even after optical activation with 30 pulses (n = 8). Here and in all optical activation experiments below, n values represent number of cells. (D) Designing an optical input (OI) for opsin activation. (Left) Single-point laser beam energy density profile of 445 nm, 5 μWatthe image plane. Experimentally a cell can be exposed to this optical input by selecting the crosshair tool (┼) as the ROI. (Right) Energy density profile of square- shaped OI area (example: 3 × 3 μm) of laser raster scan. The galvo mirrors scan the ROI at 0.87 ms/μm2 and the area of the OI determines the duration of a single pulse. (E) Optical activation at one specific wavelength and intensity (purple) is followed by imaging at a different wavelength. (F) A single HeLa cell coexpressing bOpsin-mCh and YFP-γ9 was optically activated by varying laser intensities (445 nm).
Details
-
File Typepdf
-
Upload Time-
-
Content LanguagesEnglish
-
Upload UserAnonymous/Not logged-in
-
File Pages10 Page
-
File Size-