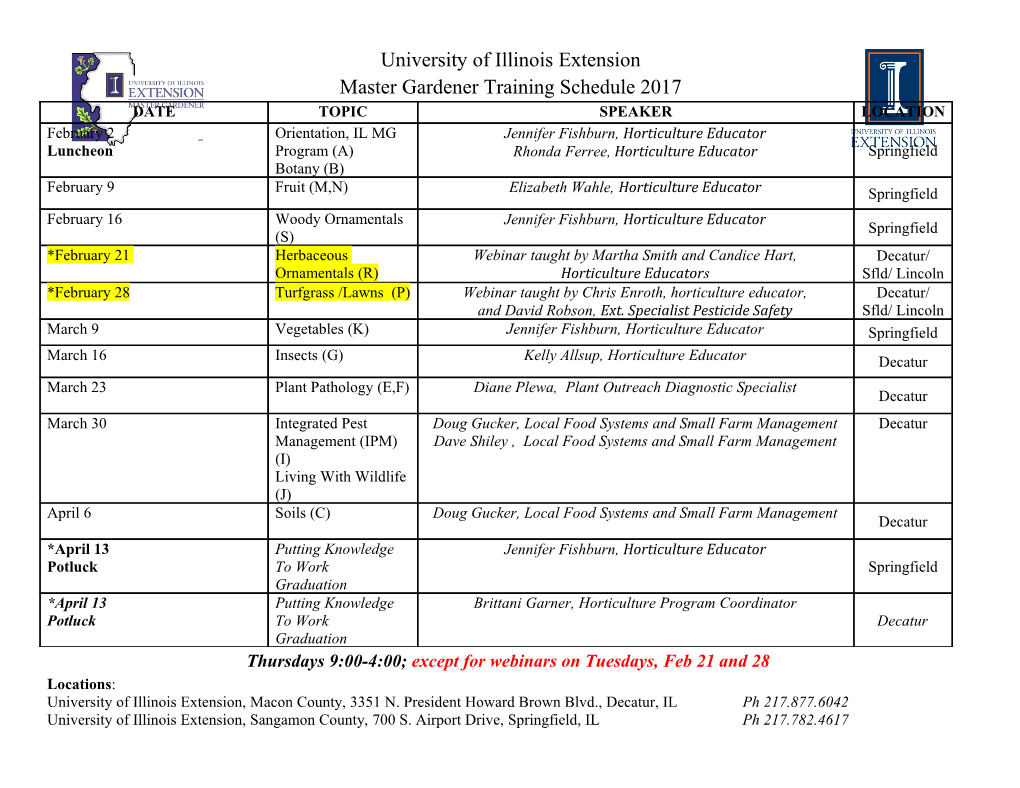
Functional characterisation of alkane-degrading monooxygenases in Rhodococcus jostii strain 8 Jindarat Ekprasert A thesis submitted to the School of Environmental Sciences in fulfilment of the requirements for the degree of Doctor of Philosophy September 2014 University of East Anglia Norwich, UK i Contents List of figures viii List of tables xiii Declaration xv Acknowledgements xvi Abbreviations xvii Abstract xxi Chapter 1 introduction 1 1.1. Significance of alkanes in the environment 2 1.1.1. Chemistry of alkanes 2 1.2. The Rhodococcus genus 3 1.2.1. Common characteristics of Rhodococcus spp. 3 1.2.2. Rhodococcus spp. are capable of degrading gaseous alkanes 4 1.2.3. Potential applications of Rhodococcus in biotechnology 5 1.3. Bacterial enzymes responsible for alkane degradation 6 1.3.1. Integral membrane, non-heme iron alkane hydroxylases (AlkB) 6 1.3.2. Soluble di-iron monooxygenases (SDIMO) 8 1.3.2.1. SDIMO classification 10 1.3.2.2. Molecular genetics of SDIMOs 13 1.3.2.3. Mutagenesis of soluble methane monooxygenase 13 1.3.3. Cytochrome P450 alkane hydroxylases 14 3.3.1. Class I P450 14 3.3.2. Class II P450 (CYP52) 15 3.3.3. Class II P450 (CYP2E, CYP4B) 15 1.3.4. Membrane bound copper-containing (and possibly iron-containing) monooxygenases 15 1.4. Alkane metabolisms in Rhodococcus spp. 16 1.4.1. Aerobic metabolism of C2-C4 gaseous alkanes in bacteria 16 1.4.1.1. Ethane (C2H6) metabolism 16 1.4.1.2. Propane (C3H8) metabolism 17 ii 1.4.1.3. Butane (C4H10) metabolism 21 1.4.2. Alcohol dehydrogenases (ADH) 22 1.4.2.1. Pyrroloquinoline quinone (PQQ) dependent alcohol dehydrogenase 23 1.4.2.2. Nicotinamide adenine dinucleotide (NAD(P)) dependent alcohol dehydrogenase 23 1.4.2.3. N,N-dimethyl-4-nitrosoaniline (NDMA) dependent alcohol dehydrogenase 24 1.5. Previous studies on mutagenesis of alkane-degrading enzymes in Rhodococcus species 25 1.5.1. The development of E. coli – Rhodococcus shuttle vectors and transformation procedures 25 1.5.2. Mutagenesis of propane monooxygenases 27 1.5.3. Mutagenesis of alkB-type alkane monooxygenases 28 Project aims 29 Chapter 2 Materials and methods 32 2.1. Bacterial strains, plasmids and primers used in this study 33 2.2. Chemicals 33 2.3. Antibiotics 33 2.4. Growth conditions 34 2.4.1. Rhodococcus jostii strain 8 35 2.4.2. Escherichia coli 36 2.5. Maintenance of bacterial strains 36 2.6. Cell dry weight measurement 36 2.7. Light microscopy 36 2.8. General purpose buffers and solutions 37 2.9. Extraction of nucleic acids 37 2.9.1. Extraction of genomic DNA from Rhodococcus jostii strain 8 38 2.9.2. Small-scale extraction of plasmids from E. coli 38 2.9.3. Extraction of RNA from Rhodococcus jostii strain 8 38 2.10. Nucleic acid manipulation techniques 38 2.10.1. DNA/RNA quantification 38 2.10.2. DNA purification 38 2.10.3. RNA purification 38 2.10.4. RNase treatment of genomic DNA 39 2.10.5. DNA restriction digests 39 iii 2.11. Polymerase chain reaction (PCR) 39 2.11.1. Design of PCR primers 39 2.12. Agarose gel electrophoresis 43 2.13. Dephosphorylation of DNA 43 2.14. DNA ligations 43 2.15. Cloning of PCR products 43 2.16. Clone library construction and restriction fragment length polymorphism (RFLP) 43 2.17. Sequencing of DNA 44 2.18. Reverse transcriptase PCR (RT-PCR) 44 2.19. Reverse transcriptase quantitative PCR (RT-qPCR) 44 2.20. Genome sequencing 45 2.21. Bacterial transformations 45 2.21.1. Preparation and transformation of chemically competent E. coli 45 2.21.2. Preparation and transformation of electrocompetent E. coli 46 2.21.3. Preparation and transformation of electrocompetent Rhodococcus jostii strain 8 47 2.22. Harvesting of cells 47 2.23. Bacterial purity checks 48 2.24. Calculation of specific growth rates 48 2.25. Preparation of cell-free extracts 48 2.26. Protein quantification 48 2.27. Sodium Dodecyl Sulfate-Polyacrylamide Gel Electrophoresis (SDS-PAGE) 49 2.28. Mass spectrometry analysis of polypeptides 50 2.29. Oxygen electrode experiments 51 2.29.1. Preparation of cell suspension 51 2.29.2. Measurement of oxygen uptake by cell suspensions in the presence of substrates 51 2.30. Enzyme assays 53 2.30.1. PQQ-dependent alcohol dehydrogenase 53 2.30.2. NAD(P)+-dependent alcohol dehydrogenase 53 2.30.3. NDMA-dependent alcohol dehydrogenase 53 2.30.4. sMMO activity using the naphthalene assay 54 Chapter 3 Growth and Oxidation studies 55 3.1. Introduction 56 3.1.1. General characteristics of Rhodococcus jostii strain 8 62 3.1.2. Cloning of SDIMO α-subunit genes of R. jostii strain 8 63 3.2. Growth profiles of R. jostii strain 8 and R. jostii RHA1 67 iv 3.3. Growth curves of R. jostii strain 8 grown on glucose and alkanes 69 3.4. Oxidation studies using the oxygen electrode 71 3.1.3. Alkane-oxidising enzymes are inducible during growth on alkanes 71 3.1.4. Oxygen consumption by whole cells in response to alcohols 73 3.1.5. Oxygen consumption by whole cells in response to other potential intermediates of alkane oxidation 76 3.5. Alcohol dehydrogenase assays 78 3.5.1. PQQ-dependent alcohol dehydrogenase assays 79 3.5.2. NAD(P)H-dependent alcohol dehydrogenases 80 3.5.3. NDMA-dependent alcohol dehydrogenases 82 3.6. Discussion and conclusion 88 Chapter 4 The genome of Rhodococcus jostii strain 8 90 4.1. Introduction 91 4.2. Genome sequencing for Rhodococcus jostii strain 8 94 4.3. Subsystems in the genome of R. jostii strain 8 94 4.4. Plasmids and transposable elements 96 4.5. The genes encoding a putative naphthalene 1, 2-dioxygenase 97 4.6. The biphenyl dioxygenase and ethylbenzene dioxygenase gene clusters 99 4.7. Analysis of propane monooxygenase gene sequences in the genome of Rhodococcus jostii strain 8 102 4.8. Analysis of alkB-type alkane hydroxylase gene sequences in the Rhodococcus jostii strain 8 genome 109 4.9. Cytochrome P450 monooxygenases in Rhodococcus jostii strain 8 114 4.10. Metabolic pathway analysis for Rhodococcus jostii strain 8 115 4.10.1. Nitrogen metabolism: reduction and fixation 116 4.10.2. Sulfur metabolism 118 4.10.3. Propanoate metabolism 120 4.10.4. Butanoate metabolism 122 4.11. Discussion and conclusions 124 v Chapter 5 Expression of propane monooxygenase and alkB-type alkane monooxygenase 126 5.1. Introduction 127 5.2. Polypeptide analysis: Expression of propane monooxygenase polypeptides during growth on propane 129 5.3. Discussion for polypeptide analysis 141 5.4. RT-qPCR: transcription of alkB and prmA in Rhodococcus jostii strain 8 grown on glucose, ethane, propane, butane and octane 145 5.4.1. RNA extraction and assessment of RNA quality 145 5.4.2. Reverse Transcriptase PCR targeting prmA and alkB 146 5.4.3. Primer optimisation for RT-qPCR 148 5.4.4. Expression of propane monooxygenase and alkane hydroxylase in R. jostii strain 8 during different growth conditions 152 5.5. Discussion for expression of prmA and alkB 158 5.6. Conclusions 160 Chapter 6 Mutagenesis of prmA and alkB in Rhodococcus jostii strain 8 161 6.1. Introduction 162 6.2. Optimisation of electroporation conditions for use in mutagenesis of prmA and alkB in R. jostii strain 8 163 6.2.1. Optimal voltage for electroporation of R. jostii strain 8 163 6.2.2. Optimal resistance for electroporation conditions of Rhodococcus jostii strain 8 165 6.2.3. Optimal competent cell culture density, recovery medium and recovery time for transformants 167 6.3. Marker exchange mutagenesis using the vector pK18mobsacB introduced by electroporation 168 6.3.1. Construction of mutants 168 vi 6.4. Introduction of mutated prmA and alkB into the chromosome of R. jostii strain 8 by electroporation 172 6.4.1. Electroporation of pAGB and pXGY 172 6.4.2. Electroporation of mutated prmA and alkB fragments 172 6.5. Screening for a prmA-deficient strain 175 6.6. Screening for alkB mutants 178 6.7. Discussion and conclusions 181 Chapter 7 Conclusions and future prospects 184 7.1. Conclusions 185 7.1.1. Growth and oxidation studies 185 7.1.2. The genome of Rhodococcus jostii strain 8 186 7.1.3. Expression studies 186 7.1.4. Mutagenesis of prmA and alkB 187 7.2. Future prospects 187 References 190 vii List of figures Figure 1.1: The crystal structure of soluble methane monooxygenase from Methylococcus capsulatus Bath 9 Figure 1.2: A phylogenetic tree showing the relationship of SDIMOs is based on alignment of 600 amino acids from the α-subunit of the hydroxylase components 11 Figure 1.3: Amino acid alignments show conserved regions of SDIMO alpha subunits targeted for PCR primer design and the expected PCR amplicon sizes from various primer combinations 12 Figure 1.4: the sMMO operon of Methylosinus trichosporium OB3b 13 Figure 1.5: Ethane metabolism by non-methane oxidising bacteria 17 Figure 1.6: The possible propane oxidation pathway by Rhodococcus rhodochrous PNKb1 19 Figure 1.7: Proposed terminal (left) ocidation and subterminal (right) of butane in butane-degrading bacteria (adapted from Arp, 1999) 23 Figure 1.8: The plasmid map of pK18mobsacB 27 Figure 3.1: Phylogenetic tree showing relationship between 6 selected isolates and their relatives 60 Figure 3.2: cell morphology of R. jostii strain 8 grown on NMS medium with 10% (v/v) propane in the headspace 62 Figure 3.3: Diagram demonstrating NVC and mmoX primer sites within the SDIMO α-subunit gene 64 Figure 3.4: Phylogenetic relationship between amino acid sequences of the putative SDIMO α-subunit from strain 8 and those from other organisms 66 Figure 3.5: Growth curves of R.
Details
-
File Typepdf
-
Upload Time-
-
Content LanguagesEnglish
-
Upload UserAnonymous/Not logged-in
-
File Pages234 Page
-
File Size-