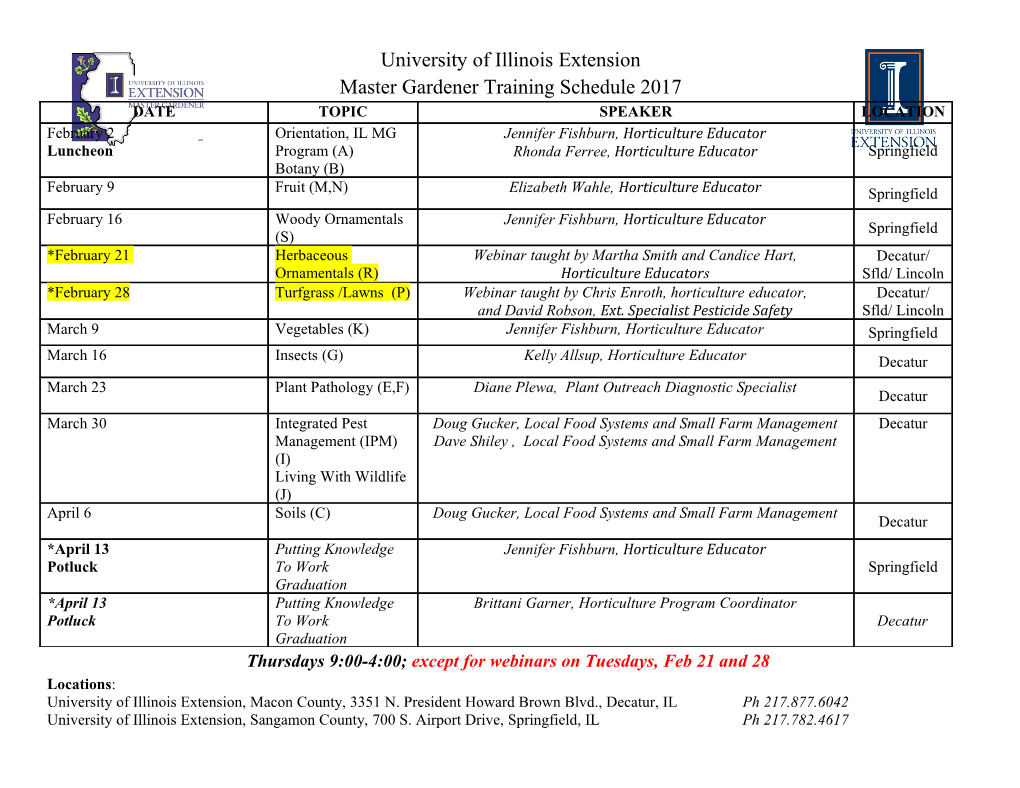
Commentary Continued evolutionary surprises among dinoflagellates Clifford W. Morden*†‡ and Alison R. Sherwood* *Department of Botany and †Hawaiian Evolutionary Biology Program, University of Hawaii, 3190 Maile Way, Honolulu, HI 96822 t is well established that chloroplasts in (also in green algae), fucoxanthin, chl c1 gene encoding the carbon assimilation Igreen and red algae are derived from a and c2 (also in stramenopiles and hapto- protein Rubisco, rbcL, has frequently primary endosymbiotic event between a phytes) and chl c1 and phycobilins (also in been used to examine deep phylogenetic cyanobacterium and a eukaryotic organ- cryptophytes), and are believed to be the relationships (12–14). However, recent ism Ϸ1 billion years ago (Fig. 1; refs. 1 and products of further endosymbioses with studies have demonstrated that there are 2). Although these two groups account for species from those groups (5–8). In this several problems associated with using many of the world’s photosynthetic spe- issue of PNAS, Yoon et al. (9) provide rbcL for this purpose. First, there appears cies, most other major taxonomic groups startling new evi- to have been ram- of photosynthetic organisms (strameno- dence that implicates pant horizontal piles—including diatoms, phaeophytes, dinoflagellate plas- gene transfer chrysophytes—and haptophytes) have tids containing fucox- Long-held theories suggested that among various al- plastids derived from a photosynthetic eu- anthin and chl c1 and primitive dinoflagellates were gal groups (14, c (derived from a 15). Reliance on karyote implying a secondary endosym- 2 heterotrophic and the addition biosis (1, 2). Still other groups, such as the haptophyte ancestor) such a gene to de- dinoflagellates, have more complicated as being ancestral to of a peridinin-containing plastid termine potential associations believed to be derived from those with peridinin. occurred relatively recently. endosymbiotic re- tertiary endosymbioses involving the en- This new paradigm in lations would be gulfment of a secondary endosymbiont. the relationship of fraught with po- Each endosymbiotic event has character- these species forces tential misinter- istic structural changes associated with it, us to rethink many aspects of dinoflagel- pretations. Second, peridinin-containing the most notable of which is the addition late evolution. dinoflagellates have a nuclear encoded of two membranes surrounding the plastid In many respects, the findings by Yoon form II Rubisco (L2 structure rather than (the inner representing the cell membrane et al. (9) allow a more parsimonious view the L8S8 structure typical of most eu- of the engulfed organism and the outer of dinoflagellate evolution. Previously karotic plastids) that is similar in sequence representing the phagocytosis vacuole long-held theories suggested that primi- homology to anaerobic bacteria (16–18). membrane) (2). Dinoflagellates, although tive dinoflagellates were heterotrophic Third, multiple copies of Rubisco have believed to be tertiary endosymbionts, and the addition of a peridinin-containing been found in some organisms (14). Al- have only 3 membranes surrounding their plastid occurred relatively recently (10), though identical or nearly identical copies plastids (1, 2), suggesting that the acqui- which was borne out by the fact that of photosynthesis related genes are often sition of too many membranes may be approximately 50% of dinoflagellate spe- found in cyanobacteria (19–21), both functionally unstable and can cause some cies are heterotrophic (1) and that many form I and form II Rubisco have been to be lost. photosynthetic dinoflagellates have re- found in some species. Dinoflagellates are fascinating organ- tained heterotrophic behavior (11). How- The genes chosen by Yoon et al. (9) were psaA (encoding the photosystem I isms that have intrigued researchers for ever, recent studies have demonstrated P700 apoprotein A1) and psbA (encoding many years. They are most well known for that photosynthetic dinoflagellates are the photosystem II thylacoid protein D1). toxic blooms associated with red tides and clearly ancestral, and that heterotrophy These genes are intimately tied to the symbiotic relationships with corals (zoox- has been independently derived numerous photosystem reaction centers and have a anthellae) (2). They contain an astound- times within the group (8). Fucoxanthin low rate of base substitution (nonsynono- ing array of unique features that has been and chl c1 and c2 are the predominant mous replacement rate K ϭ 0.022 and the impetus for continued evolutionary form of light harvesting pigments among A 0.009 for psaA and psbA, respectively; ref. studies. One is their close phylogenetic stramenopiles and haptophytes. With the 22) compared with other genes such as finding that haptophytes are the sister link with apicomplexans, organisms that rbcL (K ϭ 0.041, considered slowly evolv- group to dinoflagellates in phylogenetic A are best known for causing some of our ing; ref. 22), so that significant phyloge- analyses and that dinoflagellates with fu- most deadly infectious diseases (3, 4). netic signal should be expected even at coxanthin and chl c and c are apparently Another is the diverse array of light har- 1 2 these deep evolutionary positions. The vesting pigments within the group. Peri- ancestral to the peridinin-containing spe- resulting phylogenies were rigorously ex- dinin is a xanthophyll found exclusively in cies, there is no need to hypothesize an amined using various positions of the pro- dinoflagellates and, together with chl a independent origin of these pigments nor tein codons, amino acid sequences, and (ubiquitous among photosynthetic organ- a later endosymbiosis of a haptophyte in isms), makes up the light harvesting com- dinoflagellates. plex found in most species. Dinoflagel- Selection of the proper gene for exam- See companion article on page 11724. lates with other combinations of plastid ination of deep phylogenetic relationships ‡To whom reprint requests should be addressed. E-mail: pigments are also known, including chl b was critical for carrying out this study. The [email protected]. 11558–11560 ͉ PNAS ͉ September 3, 2002 ͉ vol. 99 ͉ no. 18 www.pnas.org͞cgi͞doi͞10.1073͞pnas.192456999 Downloaded by guest on September 26, 2021 Fig. 1. Theoretical steps of primary, secondary, and tertiary plastid endosymbiosis. (1) A nonpho- tosynthetic eukaryote engulfs a cyanobacterium. (2) The engulfed cyanobacterium becomes the cell’s plastid, and gene transfer occurs between the cyanobacterium cell and the host cell nucleus. The cyanobacterial membrane becomes the inner plas- Fig. 2. Hypothetical evolutionary relationships among algal groups and derivations of the various tid membrane and the eukaryotic vacuolar mem- dinoflagellate lineages based on possible plastid endosymbiosis events. (1) Initial symbiosis of a cyano- brane becomes the outer plastid membrane (pri- phyte/glaucophyte progenitor (see text). (2) Loss of residual peptidoglycan cell wall. (3) Loss of phycobilins mary endosymbiosis). (3) A nonphotosynthetic and gain of chl b.(4) Secondary endosymbiosis of green alga with retention of algal nucleus as nucleo- eukaryote engulfs a eukaryote with a primary plas- morph between second and third layers of plastid membrane. (5) Secondary endosymbiosis of green alga COMMENTARY tid. (4) Four plastid membranes are present from by dinoflagellate (Dinoflagellates 1). (6) Secondary endosymbiosis of red alga by cryptophyte with the primary and secondary plastids (see text); the retention of algal nucleus (nucleomorph) between second and third layers of plastid membrane. nucleus of the secondary plastid is reduced to a (7) Tertiary endosymbiosis of cryptophyte by dinoflagellate (Dinoflagellates 2). (8) Secondary endosym- nucleomorph (which is present in the chlorarach- biosis of red alga; loss of phycobilins with gain of chl c1 and c2 as light harvesting pigments; gain of niophytes and the cryptophyes); gene transfer oc- xanthophylls (predominantly fucoxanthin) as accessory pigments. (9) Tertiary endosymbiosis of diatom by curs between the nucleomorph of the secondary dinoflagellate (Dinoflagellates 3). (10) Possibly a tertiary endosymbiosis of stramenopile by haptophyte plastid and the nucleus of the host cell (secondary (see text). (11) Possibly a tertiary or quaternary endosymbiosis of haptophyte by dinoflagellate (Dinoflagellates endosymbiosis). (5) A eukaryotic cell with a second- 4) (see text and figure 3 of ref. 9 for further details). (12) Loss of fucoxanthin and chl c1 and c2 after development ary plastid is engulfed by a nonphotosynthetic eu- of peridinin as light harvesting pigment (Dinoflagellates 5). (13) Loss of plastids; roughly half of peridinin- karyote (tertiary endosymbiosis). The remnant containing dinoflagellates (Dinoflagellates 6). (14) Loss of photosynthesis in apicomplexans. nucleomorph of the secondary plastid is lost. (6)Up to six plastid membranes are theoretically possible (see text). The nucleus of the engulfed cell has been sis (9) (Fig. 2). Haptophyte and This then begs the question, how many reduced to a nucleomorph. (7) Gene transfer occurs dinoflagellate plastids, in turn, form a endosymbiotic events are required to ac- between the nucleomorph of the tertiary plastid sister group to the stramenopiles, suggest- count for all of the diversity present and the host cell nucleus. (8) The nucleomorph of the tertiary plastid is lost. Abbreviations: 1° End, ing that they were derived from a single among photosynthetic algae?
Details
-
File Typepdf
-
Upload Time-
-
Content LanguagesEnglish
-
Upload UserAnonymous/Not logged-in
-
File Pages3 Page
-
File Size-