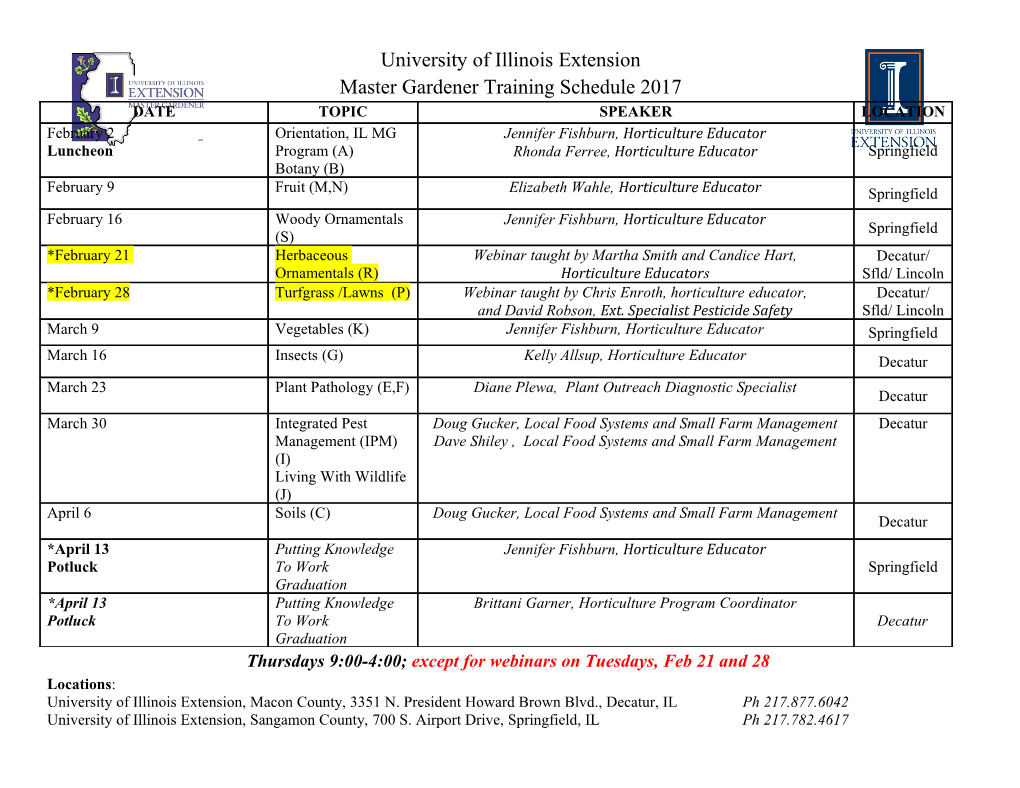
Downloaded from http://cshperspectives.cshlp.org/ on October 2, 2021 - Published by Cold Spring Harbor Laboratory Press Calcium Signaling in Synapse-to-Nucleus Communication Anna M. Hagenston and Hilmar Bading CellNetworks—Cluster of Excellence, Department of Neurobiology, and the Interdisciplinary Center for Neurosciences (IZN), University of Heidelberg, 69120 Heidelberg, Germany Correspondence: [email protected] Changes in the intracellular concentration of calcium ions in neurons are involved in neurite growth, development, and remodeling, regulation of neuronal excitability, increases and decreases in the strength of synaptic connections, and the activation of survival and pro- grammed cell death pathways. An important aspect of the signals that trigger these processes is that they are frequently initiated in the form of glutamatergic neurotransmission within dendritic trees, while their completion involves specific changes in the patterns of genes expressed within neuronal nuclei. Accordingly, two prominent aims of research concerned with calcium signaling in neurons are determination of the mechanisms governing infor- mation conveyance between synapse and nucleus, and discovery of the rules dictating trans- lation of specific patterns of inputs into appropriate and specific transcriptional responses. In this article, we present an overview of the avenues by which glutamatergic excitation of den- drites may be communicated to the neuronal nucleus and the primary calcium-dependent signaling pathways by which synaptic activity can invoke changes in neuronal gene expression programs. he significance of intracellular calcium Mauceri et al. 2011), modulation of dendritic T(Ca2þ) increases for the regulation of gene complexity (Redmond et al. 2002; Mauceri expression in neurons is well established (Bad- et al. 2011), growth factor signaling (Gall and ing et al. 1997; Chawla 2002; Greer and Green- Lauterborn 1992; Castren et al. 1998; Greenberg berg 2008; Redmond 2008; Hardingham and et al. 2009), regulation of long-term changes Bading 2010). Activity-dependent changes in in synaptic strength (Korzus et al. 2004; Lim- gene expression in neurons participate in a back-Stokin et al. 2004; Raymond and Redman broad range of processes and behaviors, includ- 2006), and memory consolidation (Bailey et al. ing synaptic activity-induced acquired neuro- 1996; Pittenger et al. 2002; Limback-Stokin protection (Hardingham et al. 2002; Papadia et al. 2004; Wood et al. 2005; Etkin et al. et al. 2005; Zhang et al. 2007b, 2009; Lau and 2006; Mauceri et al. 2011). Increases in the 2þ 2þ Bading 2009), activity-dependent regulation of intracellular concentration of Ca ([Ca ]i) synapse number, size, and function (Flavell et al. that might trigger gene transcription as a conse- 2006; Shalizi et al. 2006; Saneyoshi et al. 2010; quence of neuronal activity are mediated by Editors: Martin Bootman, Michael J. Berridge, James W. Putney, and H. Llewelyn Roderick Additional Perspectives on Calcium Signaling available at www.cshperspectives.org Copyright # 2011 Cold Spring Harbor Laboratory Press; all rights reserved. Advanced Online Article. Cite this article as Cold Spring Harb Perspect Biol doi: 10.1101/cshperspect.a004564 1 Downloaded from http://cshperspectives.cshlp.org/ on October 2, 2021 - Published by Cold Spring Harbor Laboratory Press A.M. Hagenston and H. Bading Ca2þ entry through L-type voltage-dependent Ras/MAPK cascades. The culmination of these Ca2þ channels (VDCCs) and ligand-gated chan- and other kinase cascades is the phosphoryla- nels such as N-methyl-D-aspartate receptors tion and activation of a variety of transcription (NMDARs) (Bading et al. 1993, 1995) and factors, most notably CREB, but also TCF, a-amino-3-hydroxyl-5-methyl-4-isoxazolepropi- nuclear factor kB(NF-kB), myocyte enhancer onate receptors (AMPARs) (Jonas and Burna- factor 2 (MEF2), and others (Cruzalegui and shev 1995; Cohen and Greenberg 2008), and Bading 2000; Greer and Greenberg 2008). Sev- by release of Ca2þ from the endoplasmic reticu- eral important genomic responses following lum (ER) (Fig. 1) (Hardingham and Bading neuronal activity are controlled by Ca2þ signals 1998; Power and Sah 2002, 2007; Stutzmann in the nucleus (Bading 2000; Zhang et al. 2009). 2þ et al. 2003; Watanabe et al. 2006; Hagenston For example, increases in nuclear [Ca ]i stim- et al. 2008). Ca2þ transients that invade the ulate gene transcription indirectly through the cell nucleus appear to play a particularly impor- nuclear resident Ca2þ/calmodulin-dependent tant role in activity-dependent transcription protein kinase IV (CaMKIV) (Hardingham et al. (Hardingham et al. 1997, 2001b; Zhang et al. 2001b) or directly by interaction with regula- 2007b, 2009) and are critical for several long- tors of gene transcription such as transcrip- lasting adaptive responses, including acquired tion factor downstream regulatory element neuroprotection and memory formation (Bad- antagonistic modulator (DREAM) (Carrion ing 2000; Limback-Stokin et al. 2004; Papadia et al. 1999; Ledo et al. 2002). et al. 2005; Zhang et al. 2009, 2011; Mauceri Cumulating evidence suggests that, in et al. 2011). addition to regulating transcription via its in- Molecular studies of Ca2þ-regulated geno- fluence on specific transcription factors, Ca2þ- mic responses have largely focused on the dependent modulation of genomic responses interactions between synaptic excitation, the also operates at a global level by influencing 2þ 2þ particular sources of cytoplasmic [Ca ]i in- chromatin structure. Nuclear Ca signals act- creases, the stimulation of downstream iden- ing via CaMKIV, for instance, stimulate the tified signaling cascades, and the activation or histone acetyltransferase CREB binding pro- repression of specific transcription factor tar- tein (CBP). Activated CBP, which interacts gets. Prominent among the Ca2þ sources, Ca2þ- physically with numerous transcription factors dependent second messenger cascades, and (e.g., CREB) to promote transcription, influ- transcription factors are NMDARs and L-type ences gene transcription by catalyzing histone VDCCs, Ca2þ/calmodulin-dependent protein acetylation and subsequent chromatin decon- kinase (CaMK) and Ras/mitogen activated densation (Chawla et al. 1998; Cruzalegui and protein kinase (Ras/MAPK) signaling cascades, Bading 2000; Alarcon et al. 2004; Korzus et al. and the transcription factor cyclic AMP 2004; Bedford et al. 2010). In a similar vein, response element binding protein (CREB) as nuclear Ca2þ signals induce the subcellular well as the ternary complex factor (TCF)/serum redistribution of class II histone deacetylases response factor (SRF) transcription factor (HDACs), enzymes whose activity leads to complex (Fig. 2) (Bading et al. 1993; Xia et al. chromatin compression and diminished acces- 1996; Cruzalegui and Bading 2000; Greer and sibility by several transcription factors to their Greenberg 2008). Briefly summarized, synapti- target binding sequences (Chawla et al. 2003; cally released glutamate binds to and activates Tian et al. 2009, 2010b). Recent results indicate NMDARs and AMPARs, leading to a depolari- that also de novo DNA methylation, a mecha- zation of the postsynaptic membrane and the nism stimulating global chromatin remodeling activation of L-type VDCCs. Ca2þ influx and gene transcription, is controlled by a neuro- through synapse-associated dendritic NMDARs nal activity and Ca2þ-dependent mechanism and L-type VDCCs is sensed by calmodulin (Chen et al. 2003; Martinowich et al. 2003; (CaM), which initiates a chain of signaling Zhou et al. 2006; Skene et al. 2010). Ca2þ- events including stimulation of CaMK and dependent synapse-to-nucleus communication 2 Advanced Online Article. Cite this article as Cold Spring Harb Perspect Biol doi: 10.1101/cshperspect.a004564 Downloaded from http://cshperspectives.cshlp.org/ on October 2, 2021 - Published by Cold Spring Harbor Laboratory Press Neuronal Ca2þ Signaling to the Nucleus glu Ca2+ PIP2 mGluR NMDAR EAAT Plasma membrane DAG VDCC (2) (1) gq/11 (3) PLC (4) IP3 ER IP3R RyR Cytosol Nucleus (5) Figure 1. Sources of synaptic activity induced calcium signals. Here we consider five distinct routes by which Ca2þ may enter the neuronal cytoplasm. (1) Ca2þ may enter the cell from the extracellular space via ionotropic gluta- mate receptors, particularly NMDARs. (2) Ca2þ may also pass from the extracellular space into the cytoplasm by way of VDCCs, most notably the dihydropine-sensitive class of high voltage-activated, or L-type, VDCCs. (3) Stimulation of mGluRs can trigger release of Ca2þ into the cytoplasm from intracellular Ca2þ stores like ER: syn- aptically released glutamate activates mGluRs, which are coupled via Gq/11 GTP-binding proteins to PLC. Acti- vated PLC cleaves membrane-bound PIP2 to yield DAG and soluble IP3, which may then diffuse to and activate 2þ IP3Rs on the ER membrane. (4) Cytosolic Ca signals originating from any of the ligand-gated glutamate recep- 2þ tors, VDCCs, or from IP3Rs can be amplified via the Ca -dependent activation of RyRs and subsequent internal release of Ca2þ from intracellular stores. (5) Synaptically released glutamate may activate mGluRs on the inner nuclear envelope subsequent to being taken up by EAATs on the plasma membrane first into the cytosol, and then by EAATs on the nuclear envelope into the nuclear lumen. Stimulation of intranuclear mGluRs may conse- 2þ quently lead to the release of Ca directly into the nucleus from IP3Rs localized on the inner nuclear envelope. therefore involves
Details
-
File Typepdf
-
Upload Time-
-
Content LanguagesEnglish
-
Upload UserAnonymous/Not logged-in
-
File Pages33 Page
-
File Size-