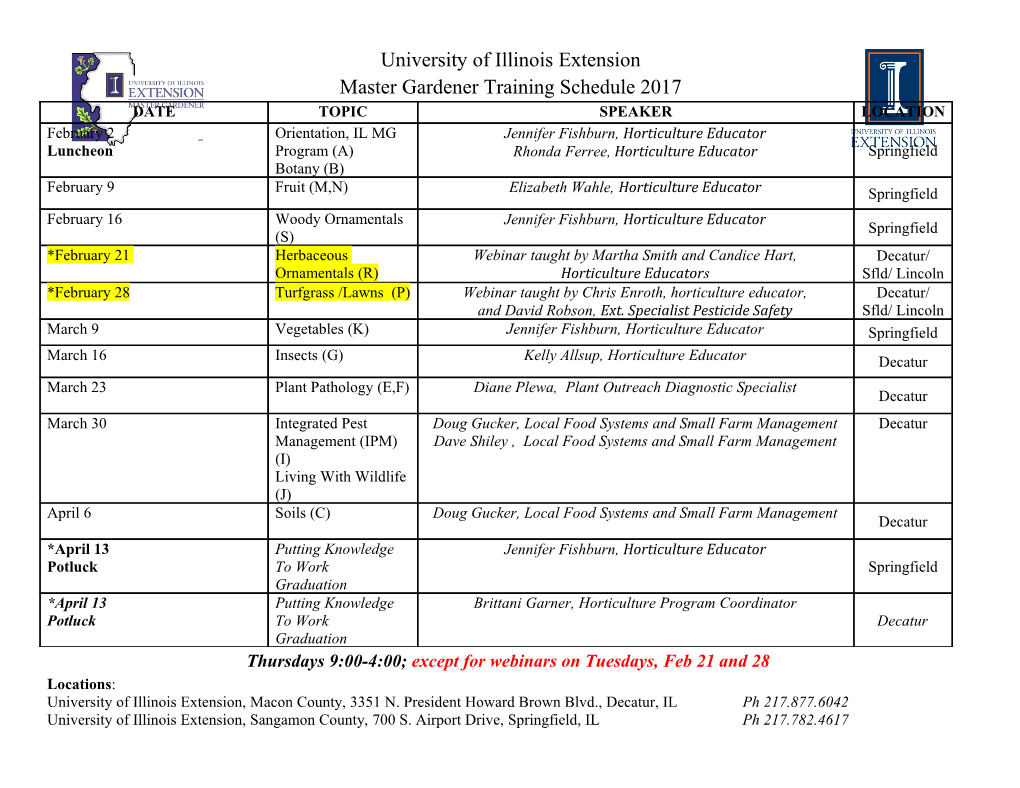
Chapter 1 - Introduction 1 1.1 Epigenetic modifications and gene silencing The fact that a multicellular organism is able to undergo cellular differentiation, even though every cell contains the same genetic material, indicates the existence of an extra layer of phenotype-determining factors. Cellular differentiation is associated with the development of different patterns of gene expression in individual cells or groups of cells, and the way in which these patterns are established involves epigenetics. Epigenetics refers to the process by which transcriptional states are changed in a relatively permanent way in the absence of DNA mutation. Epigenetic gene silencing or activation results from modifications to the gene in question either by changing the methylation state of the DNA or by modifying the proteins that package the DNA. There is increasing evidence that in some cases RNA molecules are also involved. Epigenetic modifications are generally erased and reset between generations in order to provide the necessary pluripotent starting state from which to begin development in the next generation. 1.1.1 DNA methylation DNA methylation is the best studied epigenetic modification. In mammals DNA methylation is always associated with cytosine residues, generally occurring at CpG dinucleotides in a symmetrical fashion, where the cytosines on both strands are methylated. Methylation of cytosine involves the transfer of a methyl group from an S-adenosyl-L-methionine to the 5-position of cytosine, resulting in the modified base 5-methylcytosine (Jeltsch, 2002) (Figure 1.1). Estimates of methylation levels at CpGs in mammals range from 50-90% (Gruenbaum et al., 1981; Jeltsch, 2002). DNA 2 hypermethylation at promoters usually correlates with transcriptional repression and gene silencing. cytosine 5-methylcytosine NH NH2 2 CH3 N DNA methyltransferase N O N O N H H HO NH 2 HO NH2 O + O S S O O CH N N 3 N N NH2 NH HO HO 2 OH N OH N N N S-adenosyl-L-methionine S-adenosyl-L-homocysteine Figure 1.1 Conversion of cytosine into 5-methylcytosine. A DNA methyltransferase enzyme transfers a methyl group (blue circle) from S-adenosyl-L-methionine to the 5-position of cytosine, producing 5-methylcytosine. CpG dinucleotides tend to be clustered in specific regions termed CpG islands. These are generally associated with the promoter regions of genes and tend to be hypomethylated and transcriptionally active. The remainder of the genome is CpG sparse and this is thought to be due to the frequent transition of 5-methylcytosine to thymine via spontaneous deamination (Duncan and Miller, 1980). The CpGs in the islands are thought to be protected from deamination because they are not methylated. DNA methylation patterns are maintained mitotically by DNA methyltransferase 1 (Dnmt1). This enzyme recognises hemi-methylated DNA substrates following DNA 3 replication, and copies the methylation state onto the newly synthesized strand (Leonhardt et al., 1992). De novo methylation is performed by the two methyltransferases Dnmt3a and Dnmt3b, which are required for the establishment of DNA methylation in early development (Okano et al., 1999). All methyltransferases are essential for normal mammalian development. Homozygous knockouts of Dnmt1 show embryonic lethality around mid-gestation (Li et al., 1992). Dnmt3a and Dnmt3b show an overlap in function during early embryogenesis; homozygous knockouts of Dnmt3a die within a few weeks of birth, homozygous knockouts of Dnmt3b develop normally until approximately 9.5 days post coitum (dpc) but later show developmental defects and embryonic lethality, compound homozygous knockouts of Dnmt3a and Dnmt3b die before 11.5 dpc with more severe embryonic defects than either single Dnmt3a or Dnmt3b homozygous knockouts (Okano et al., 1999). A large proportion of DNA methylation in mammals is associated with retrotransposons and other transposable elements, and so it has been suggested that DNA methylation is involved in genome defence (Yoder et al., 1997). Transposable elements are very common in the mammalian genome, with estimates predicting up to 45% of the genome being transposable element derived (Jordan et al., 2003), although much of this is non-functional due to mutation. Some elements however remain transcriptionally active and some can even undergo retrotransposition. Integration of transposable elements can result in the production of transposon-derived transcripts and subsequent proteins that could be deleterious to the host. Transposable elements can take over control of endogenous genes by donating cis-regulatory sites, leading to aberrant expression or the production of mutant transcripts (Druker et al., 2004; Hatada et al., 2003; Ukai et al., 2003). As a result, in a wide variety of organisms 4 transposable elements become heavily methylated once they integrate into the host genome, and this is associated with transcriptional silencing (Cambareri et al., 1996; Lavie et al., 2005). In confirmation of this fact, mouse embryos deficient in Dnmt1 show increased retroviral transcriptional activity (Gaudet et al., 2004; Walsh et al., 1998). While DNA methylation is an important epigenetic process in development and gene silencing, there continues to be much debate about whether it is causative or a consequence of the silencing. There is increased awareness that changes to the chromatin packaging the DNA also plays a significant role. 1.1.2 Histone modifications Post-translational modifications to histone proteins are associated with changes to chromatin structure, resulting in transcriptionally active or inactive chromatin states. These modifications involve covalent additions of small molecules to the amino- terminal tail of the histone protein. Histone acetylation Acetylation of histones occurs at lysine residues, predominantly on histones H3 and H4. Acetylation is associated with a transcriptionally active chromatin configuration. When the lysine residues are acetylated the overall positive charge of the histone protein is neutralised, resulting in a weakening of charge interactions with the DNA molecule (Allfrey, 1966). Histone acetylation also influences histone-histone and histone-regulatory protein interactions (Luger and Richmond, 1998). All these 5 interactions lead to an open chromatin configuration that is more accessible to transcription factors. Histone acetylation is performed by histone acetyltransferases (HATS) of which there are several families. The main HATS in the mouse are grouped in the GNAT (GCN5- related N-acetyltransferases) family and the MYST (named after its founding members: MOZ, Ybf2/Sas3, Sas2, Tip60) family, although there are other HATS such as Crebbp (CREB-binding protein) and Ncoa1 (nuclear receptor coactivator 1) (Marmorstein and Roth, 2001; Thomas et al., 2007). Histone deacetylation is performed by histone deacetylases (HDACS), of which 11 (Hdac1-11) have been identified in the mouse. Having both acetyltransferases and deacetylases present means that the acetylation of histones is in constant flux and can be tightly regulated. Histone methylation Methylation of histones occurs at lysine residues of histones H3 and H4. There is no simple correlation between histone methylation and transcriptional activity, since the latter is dependent upon which lysine residue is methylated and whether that residue is mono-, di- or tri-methylated. A good example of the specificity associated with individual lysine residues and their methylation state is the behaviour of H3Lys4. Di-methylation of H3Lys4 is associated with both active and inactive euchromatic regions, while tri-methylation is present exclusively at active genes. In the case of H3Lys9 however, both di- and tri- methylation are associated with transcriptionally inactive heterochromatin, with the 6 methyl moiety on H3Lys9 able to recruit heterochromatin protein 1 (HP1) (Bannister et al., 2001; Lachner et al., 2001). Both histone methyltransferases (HMTases) and demethylases have been identified in mammals. Histone methyltransferases are SET (Su(var)3-9, Enhancer-of-zeste, Trithorax) domain-containing proteins that show different specificities for different lysine residues. For example, Suv39h1 and h2 appear to only methylate H3Lys9, whereas G9a can methylate H3Lys9 and H3Lys27 (Tachibana et al., 2001). Histone demethylases have only recently been discovered. Lysine-specific demethylase 1 (LSD1) is a histone demethylase, and inhibition of this protein leads to increased levels of H3Lys4 methylation and increased gene activity (Huang et al., 2007). Other demethylases include the JMJD2 subfamily (part of the Jumonji C domain-containing family), which consists of four unique demethylases that have different activities. JMJD2A and C demethylate di- and tri-methylated H3Lys9 and H3Lys36, whereas JMJD2D demethylates mono-, di- and tri-methylated H3Lys9 (Shin and Janknecht, 2007). Other histone modifications Apart from histone acetylation and methylation, there are several other covalent modifications that can occur to histone tails. Phosphorylation of H3Ser10 has been linked to chromatin condensation and possibly gene expression (Johansen and Johansen, 2006). Ubiquitination of histone H2A is associated with the transcriptionally silent XY body (a specialised chromatin territory containing the transcriptionally repressed sex chromosomes) in males and the inactive X in females (Baarends et al., 2005). Sumoylation of histone H4 is thought to repress transcription 7 through the recruitment of HDACS and HP1 (Shiio and Eisenman, 2003).
Details
-
File Typepdf
-
Upload Time-
-
Content LanguagesEnglish
-
Upload UserAnonymous/Not logged-in
-
File Pages147 Page
-
File Size-