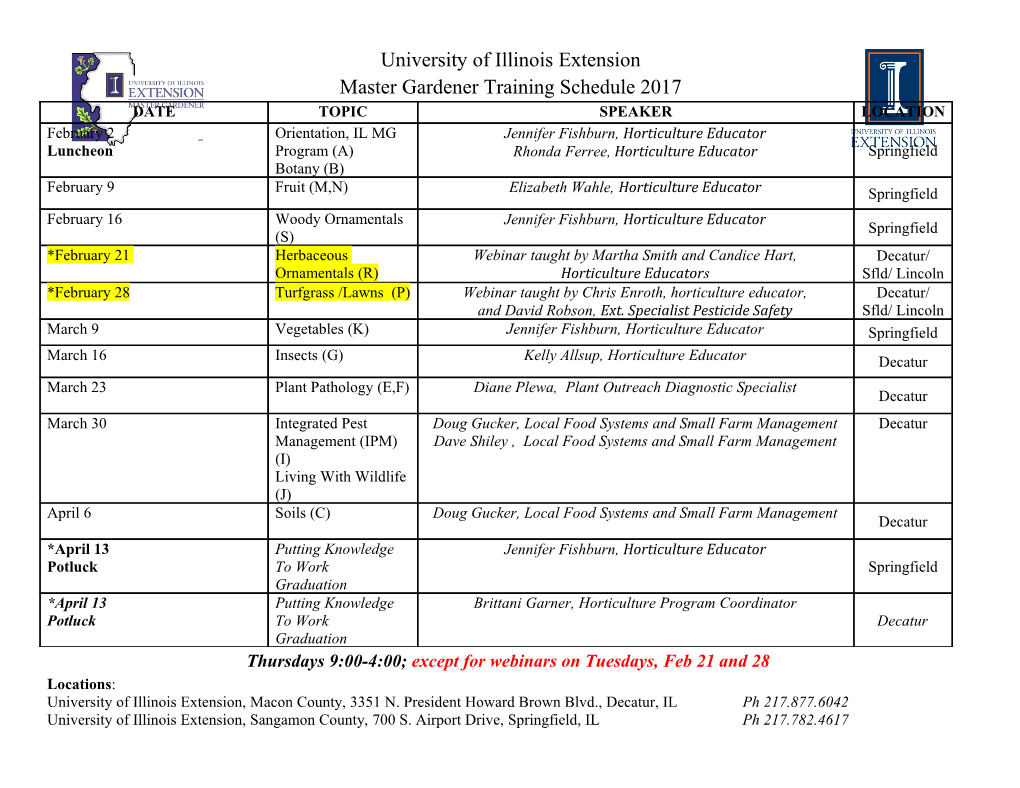
J. Cell Sci. 39, 29-52 (1979) 29 Printed in Great Britain © Company of Biologists Limited TEMPERATURE EFFECTS ON ANAPHASE CHROMOSOME MOVEMENT IN THE SPERMATOCYTES OF TWO SPECIES OF CRANE FLIES {NEPHROTOMA SUTURALIS LOEW AND NEPHROTOMA FERRUGINEA FABRICIUS) CATHERINE J. SCHAAP AND ARTHUR FORER Biology Department, York University, Dovmsview, Ontario MJj, 1P3, Canada SUMMARY Using phase-contrast cinemicrography on living crane fly (Nepkrotoma suturalis Loew and Nephrotoma ferruginea Fabricius) spermatocytes, we have studied the effects of a range of temperatures (6-30 °C) on the anaphase I chromosome-to-pole movements of both autosomes and sex chromosomes. In contrast to previous work we have been able to study chromosome-to- pole velocities of autosomes without concurrent pole-to-pole elongation. In these cells we found that the higher the temperature, the faster was the autosomal chromosome movement. From reviewing the literature we find that the general pattern of the effects of temperature on chromosome movement is similar whether or not pole-to-pole elongation occurs simultaneously with the chromosome-to-pole movement. Changes in cellular viscosities calculated from measurements of particulate Brownian movement do not seem to be able to account for the observed velocity differences due to temperature. Temperature effects on muscle contraction speed, flagellar beat frequency, ciliary beat frequency, granule flow in nerves, and chromosome movement have been compared, as have the activation energies for the rate-limiting steps in these motile systems: no distinction between possible mechanisms of force production is possible using these comparisons. The data show that even the different autosomes within single spermatocytes usually move at different speeds. These velocity differences cannot simply be related to chromosome size as the autosomes are visually indistinguishable. The sex chromosomes start their anaphase poleward movement after that of the autosomes, and move more slowly (by a factor of about 4), but their velocities appear to be affected by temperature in the same fashion as those of the autosomes. The interval between the onset of autosome anaphase and sex chromosome anaphase is also affected by temperature: the higher the temperature, the shorter the interval between the 2 stages. We have observed abnormalities in sex chromosome segregation, which may be due to temperature, but have not determined what the exact temperature shift conditions are that cause these abnormalities. INTRODUCTION The mechanisms which produce the movement of chromosomes during anaphase are still unknown, and the hypotheses are subject to much debate (for reviews see Schrader, 1953; Gruzdev, 1972; Nicklas, 1975). Anaphase movement can be sub- divided into 2 distinct processes which may or may not occur simultaneously: Send correspondence to C. J. Schaap. 3 CEL39 30 C.J. Schaap and A. Forer chromosome-to-pole movement and spindle pole-to-pole elongation. These 2 pro- cesses may have different mechanisms (e.g. Ris, 1949; Oppenheim, Hauschka & Mclntosh, 1973; McDonald, Pickett-Heaps, Mclntosh & Tippit, 1977). To under- stand better the mechanisms of anaphase chromosome movement, workers have tried to alter chromosome-to-pole movement, or pole-to-pole elongation, or both, by means of experimental treatments including altered temperature, increased pressure, addition of drugs, ultraviolet microbeam irradiation, and micromanipulation of chromosomes (e.g. Inoue", 1952; Taylor, 1959; Inoue", 1964; Forer, 1965; Nicklas, 1973; Oppenheim et al. 1973; Inoue & Ritter, 1975; Salmon, 1976). These studies have led to the formulation of several models for anaphase chromosome movement (e.g. Mclntosh, Hepler & Van Wie, 1969; Bajer, 1973; Forer, 1976; Inoue, 1976; Margolis, Wilson & Keifer, 1978). Each model has faults, however, and there is no universally accepted model for anaphase chromosome movement. This paper represents the first part of a study of the effects of temperature on anaphase chromosome movement in the first meiotic division of spermatocytes of crane flies {Nephrotoma suturalis and Nephrotoma ferruginea). There are several factors which make these animals suitable for studies of anaphase: (1) there are only 3 pairs of autosomes in large cells, making it possible to follow the movement of indivi- dual chromosomes; and (2) the major part of the chromosome-to-pole movement precedes the pole-to-pole elongation (Forer, 1964, 1965, 1966). Thus we are able to measure the effect of temperature on chromosome-to-pole movement separately from the effect of temperature on pole-to-pole elongation, which was not possible in previous studies (Barber, 1939; Ris, 1949; Fuseler, 1973, 1975). In this paper we report the effects of a range of temperatures on anaphase velocities in crane-fly spermatocytes: anaphase chromosome-to-pole velocities tend to increase with tem- perature within the range studied (6-30 °C) and at any given temperature chromo- somes in N. suturalis spermatocytes tend to move faster than those in N. ferruginea spermatocytes. MATERIALS AND METHODS Animals and living spermatocyte cell preparations The 2 species of crane flies (Nephrotoma suturalis Loew and Nephrotoma ferruginea Fabricius) used in these experiments are reared in the laboratory, using methods described in detail in Forer (1964). The N. suturalis stock originated from North Carolina, while the N. ferruginea stock originated from Toronto. Under our conditions spermatocytes enter prometaphase of the first meiotic division about 10-12 days after the male larvae enter the fourth instar stage. The testes are dissected out of the appropriate larvae while the larvae are immersed under oil (Halocarbon Oil series 10—25: Halocarbon Products Corporation, Hackensack, New Jersey, U.S.A.). The testes are then transferred to a drop of Voltalef oil (Huile 10 S: Ugine Kuhlmann, Division Plastiques; II, bd. Pershing, 75017-Paris), where the surrounding fat is carefully dissected away. (In this transfer and all subsequent steps, caution is exercised to avoid exposure of the testes to the air.) Testes are then placed under Voltalef Huile 10 S on the bottom of a holder designed to fit the temperature-control slide. The testes are punctured and the cells spread out onto the glass surface of the bottom of the holder. After the temperature-control slide is mounted on the microscope stage, the preparation is scanned and a flat cell in metaphase I is located. In our experiments, all cells were at the experi- Temperature and anaphase movement 31 mental temperature at least 10 min before the start of anaphase. Cells were photographed from metaphase until at least the end of anaphase. Pictures were taken at intervals of 20—60 s depending on the temperature (e.g. 60 s at 10 °C or 20 a at 25 °C). Temperature-control slide and supporting apparatus The temperature-control set up is similar to that used by Stephens (1973). Modifications to the Stephens system were however necessary because we used an inverted microscope. We used a Zeiss 40 x (N.A. 075) phase-contrast water-immersion lens with a long working distance. To control the temperature of the coolant, 50 % ethanol, we used a Lauda K2/RD (Brinkmann Instruments) constant temperature circulator: this both brings the solution to the proper temperature and circulates it. Thermistor probes (Teflon Probe, Part no. 44104) con- nected to a YSI Tele-Thermometer (Model 43 TZ Yellow Springs Instrument Co., Inc., Yellow Springs, Ohio, U.S.A.) were used to monitor the temperature of coolant going into and coming out from the temperature-control slide. When care was taken to dislodge air bubbles from the thermistors, the incoming and outgoing temperatures differed by only about 0-5 °C or less. The Lauda circulator temperature varied by only ±o-oi to ±0-03 °C during the course of an experiment. Time-lapse apparatus and photography The experiments were recorded on 16-mm film (Kodak Plus X Negative Film 7231). We used a Bolex (H-16-J) with a time-lapse camera drive controlled by a time-lapse control panel (R. J. Matthias and Associates, Houston, Texas, U.S.A.). The negatives were developed with Diafine in a Cramer automatic processor (Sarasota, Florida, U.S.A.). Positive working prints were made at a commercial laboratory. Film analysis and statistics The films were analysed frame by frame using an Athena projector (Model 224 by L-W Photo Inc., Van Nuys, California, U.S.A.). The working prints were projected onto a table via a mirror on a retort stand in front of the projector. For the analysis the final magnification of the cells was 2000 times. The magnification of the film was calibrated using photographs of a stage micrometer. To determine the velocities of the separating autosomal chromosome pairs, we measured interkinetochore distances parallel to the pole-to-pole axis in successive frames of the film as anaphase proceeded. These measurements were plotted with respect to time, and a typical result is given in Fig. 1. The chromosome separation velocity was determined by standard least mean squares linear regression line (Sokal & Rohlf (1969), p. 419) through the points from the linear portion of the distance-v.-time graph (e.g. the points between the arrows in Fig. 1). To determine the velocities by which the sex chromosomes separate the distance between the farthest kinetochores was measured in successive frames. Distance-v.-time graphs were plotted and the slopes of the initial steep parts of the graphs were calculated by linear regression. The slopes of the distance-v.-time curves are the velocities at which the autosomes or sex chromosomes separate. These will be referred to as the chromosome velocities in the results. Since there is no pole-to-pole elongation until at least the end of autosomal anaphase, the chromosome velocities calculated for autosomes are directly proportional to the chromosome-to- pole velocities. Since the 2 separating autosome half-bivalents usually move at the same speed, then their velocities to the poles are half the calculated autosomal chromosome velocities.
Details
-
File Typepdf
-
Upload Time-
-
Content LanguagesEnglish
-
Upload UserAnonymous/Not logged-in
-
File Pages24 Page
-
File Size-