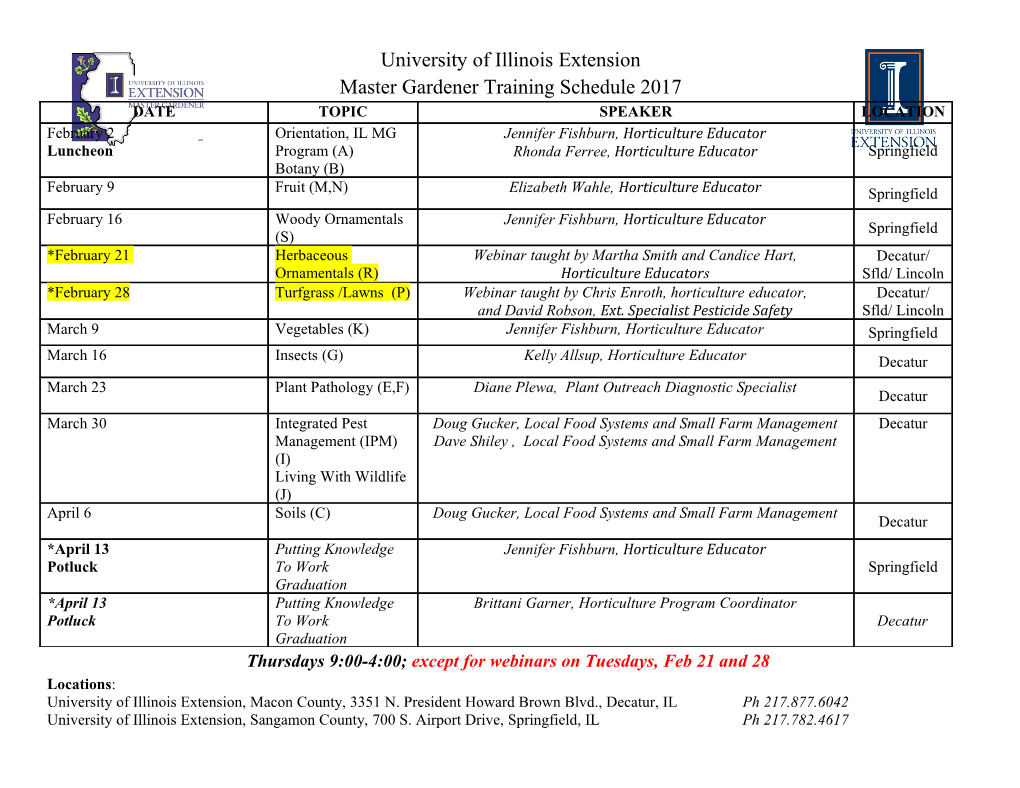
Moderate Bioclogging Leading to Preferential Flow Paths in Biobarriers by Katsutoshi Seki, Martin Thullner, Junya Hanada, and Tsuyoshi Miyazaki Abstract Permeable reactive barriers (PRBs) are an alternative technique for the biological in situ remediation of ground water contaminants. Nutrient supply via injection well galleries is supposed to support a high microbial activity in these barriers but can ultimately lead to changes in the hydraulic conductivity of the biobarrier due to the accumulation of biomass in the aquifer. This effect, called bioclogging, would limit the remediation efficiency of the biobarrier. To evaluate the effects bio- clogging can have on the flow field of a PRB, flow cell experiments were carried out in the laboratory using glass beads as a porous medium. Two types of flow cells were used: a 20- 3 1- 3 1-cm cell simulating a single injection well in a one- dimensional flow field and a 20- 3 10- 3 1-cm cell simulating an injection well gallery in a two-dimensional flow field. A mineral medium was injected to promote microbial growth. Results of 9 d of continuous operation showed that conditions, which led to a moderate (50%) reduction of the hydraulic conductivity of the one-dimensional cell, led to a preferential flow pattern within the simulated barrier in the two-dimensional flow field (visualized by a tracer dye). The bioclogging leading to this preferential flow pattern did not change the hydraulic conductivity of the biobarrier as a whole but resulted in a reduced residence time of water within barrier. The biomass distribution measured after 9 d was consistent with the observed clogging effects showing step spatial gradients between clogged and unclogged regions. Introduction advantage of PRBs compared to other remediation tech- The efficiency optimization of in situ ground water niques is that after installation of the barrier only limited remediation as well as the minimization of costs and risks activities are required, e.g., periodical injection of reactive of such remediation measures is an active field of research material and monitoring of performance. Thus, the longev- for science and engineering. Among other techniques, e.g., ity of a PRB is an important issue for the efficiency of this pump-and-treat or monitored natural attenuation, perme- remediation technique. There are two main factors leading able reactive barriers (PRB) have been proposed for the in to a reduced PRB efficiency: first, the reactivity of the situ remediation of contaminated ground water. The basic reactive material can become exhausted, and second, the principle of this technique is to install a reactive zone permeability of the barrier can not be maintained, i.e., con- downgradient of the source of contamination. Until now, taminants can bypass the reactive zone without being the majority of PRBs are based on the abiotic degradation degraded. of contaminants using variable reactive media depending Processes potentially reducing the long-term perme- on the target contaminants (Scherer et al. 2000). However, ability of a PRB include the physical (e.g., particle deposi- during the past years there has been a considerable increase tion), chemical (e.g., mineral precipitation), and biological in studies focusing on engineered PRBs making use of the (e.g., biomass buildup or microbial gas production) clog- microbial remediation of contaminants (Kalin 2004). Tech- ging of the reactive zone. The latter process is of increased niques to construct a PRB depend on the reactive material relevance for microbial PRBs as the buildup of a sufficient used as well as on the site conditions. Microbial PRBs were amount of biomass is a necessary prerequisite for the bio- either installed by excavating parts of the aquifer and refill- remediation of the contaminants. Microbially induced ing the reactive material (Gavaskar 1998), or by installing clogging has been observed in many natural or artificial well arrays, which supply substrates supporting microbial porous media (Baveye et al. 1998), e.g., at the bottom of growth and activity (e.g., Dybas et al. 2002). The main waste water infiltration ponds (Davis et al. 1973), at septic tank effluents (Kristiansen 1981), or in the vicinity of ground water discharge wells (van Beek and van der Kooij Copyright ª 2006 The Author(s) 1982). More relevant for PRBs, the injection of substrates, Journal compilation ª 2006 National Ground Water Association. which promote microbial growth in an aquifer, is known to 68 Ground Water Monitoring & Remediation 26, no. 3/ Summer 2006/pages 68–76 clog waste water injection wells (e.g., Oberdorfer and Peterson 1985) or artificial recharge wells (e.g., Rebhun and Schwarz 1968; Vecchioli 1970). Furthermore, injecting growth- promoting substrates into an aquifer in order to enhance the bioremediation of contaminants can also lead to micro- bially induced clogging of the vicinity of the well, which reduces the efficiency of this well significantly (McCarty et al. 1998). In contrast, injecting growth-promoting sub- strates into an aquifer via well galleries is a technique to establish impermeable subsurface barriers making use of the microbial clogging—or bioclogging effect (Trefry et al. 1998; Hiebert et al. 2001). Therefore, bioclogging might also occur around well galleries used to install microbial PRBs, severely challenging their remediation performance. Unfortunately, only little is known on bioclogging of PRBs under field conditions because controlled experiments that address the significance and extent of such phenomena on the hydrodynamic characteristics of PRBs are lacking (Scherer et al. 2000). In contrast, bioclogging has been investigated in sev- eral laboratory studies showing that in columns or flow cells with a one-dimensional flow field the growth of biomass can lead to severe reductions of hydraulic conductivity of the system (e.g., Taylor and Jaffe´ 1990; Cunningham et al. 1991; Vandevivere and Baveye 1992a; Seki et al 1998). Furthermore, it has been shown in flow cells having a two- Figure 1. Setup of flow cell A (top) and flow cell B (bottom). dimensional flow field that bioclogging in the vicinity of an injection well can lead to significant changes in the flow field causing a flow bypass of the clogged zone without thickness 1 cm) simulating a two-dimensional flow field. changing the average hydraulic conductivity of the entire Both flow cells were made of light transparent acrylic plas- system (Kildsgaard and Engesgaard 2002; Thullner et al. tic material and were uniformly packed with glass beads 2002a). Extrapolating the latter observations to the field of 0.1-mm mean diameter resulting in a bulk density of scale implies that bioclogging might limit the performance 1.59 Mg/m3, which corresponds to total porosity of 0.383. of a PRB without necessarily being recognized by the moni- Inlet and outlet of the flow cells were supported by 0.1-mm toring system of the PRB. nylon mesh screens. The inflow and the outflow of the flow Up to now, an explicit comparison with conditions lead- cells (one port each for flow cell A and three ports each for ing to the bioclogging of a column and conditions leading flow cell B) were kept at a constant pressure head with to the flow bypass of a bioclogged zone has not been a head difference between inlet and outlet of 6 cm resulting done. Addressing this question would help verify the abil- in an average hydraulic gradient along the flow cells of 0.3. ity of predicting the risk for bioclogging occurring in Additional injection ports were installed in flow cell A at a PRB based on the results of laboratory studies. Further- 4 cm distance (tracer injection port) and in flow cells A and more, the investigation of a clogged laboratory-scale bio- B at 10 cm distance from the inlet (nutrient injection ports). barrier would help to determine criteria, which can be used At the bottom of both flow cells, water manometers were to determine the occurrence of bioclogging in the field. installed for monitoring the local distribution of the pres- The objective of this study is to investigate the condi- sure heads. The manometers were located at 4, 7, 10, 13, tions leading to a bioclogging-induced flow bypass of an and 16 cm from the inlet. Flow cell A was equipped with injection well. Results on the bioclogging in flow cells one row of manometers and one nutrient injection port, with one-dimensional and with two-dimensional flow fields whereas flow cell B was equipped with three parallel rows were compared to clarify to what extent the processes in of manometers (with 3-cm distance between each manome- a two-dimensional flow field, typical for a biobarrier, might ter row and 2-cm distance between the outer manometers be predicted based on column experiments. and the wall of the flow cell; Figure 1 bottom) and three tracer and nutrient injection ports each. Each flow cell was operated under water-saturated con- Materials and Methods ditions, in horizontal position, and at a constant tempera- ture of 20°C. After packing, the inoculum was injected Setup and Operation of the Flow Cells into the nutrient injection ports. This injection lasted until Experiments were conducted in two different flow a 2-cm region around the injection ports was inoculated cells. Flow cell A (Figure 1 top: length 20 cm; width 1 cm; using Brilliant Blue FCF dye in the inoculum to determine thickness 1 cm) simulating a one-dimensional flow field the inoculated area visually. After 24-h incubation time, and cell B (Figure 1 bottom: length 20 cm; width 10 cm; continuous flow was started. Deionized water was supplied K. Seki et al./ Ground Water Monitoring & Remediation 26, no. 3: 68–76 69 to the inlet ports, and the mineral medium was supplied to Results the nutrient injection ports using Mariotte bottles, both kept at the same pressure head.
Details
-
File Typepdf
-
Upload Time-
-
Content LanguagesEnglish
-
Upload UserAnonymous/Not logged-in
-
File Pages9 Page
-
File Size-