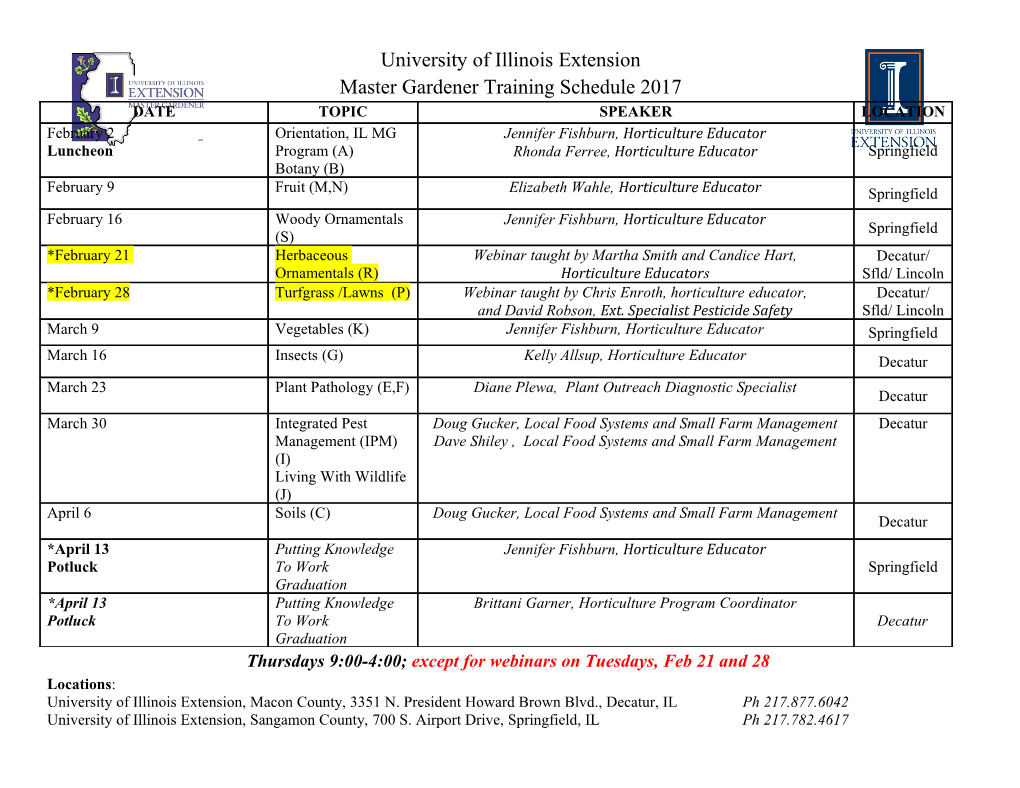
Atmos. Chem. Phys., 17, 6477–6492, 2017 www.atmos-chem-phys.net/17/6477/2017/ doi:10.5194/acp-17-6477-2017 © Author(s) 2017. CC Attribution 3.0 License. Surface ozone in the Southern Hemisphere: 20 years of data from a site with a unique setting in El Tololo, Chile Julien G. Anet1,2, Martin Steinbacher1, Laura Gallardo3,4, Patricio A. Velásquez Álvarez5,6, Lukas Emmenegger1, and Brigitte Buchmann1 1Laboratory for Air Pollution/Environmental Technology, Swiss Federal Laboratories for Materials Science and Technology Empa, Duebendorf, Switzerland 2WSL Institute for Snow and Avalanche Research SLF, Davos, Switzerland 3Departamento de Geofísica de la Universidad de Chile, Blanco Encalada 2002, piso 4, Santiago, Chile 4Center for Climate and Resilience Research (CR2), Blanco Encalada 2002, Santiago, Chile 5Dirección Meteorológica de Chile, Av. Portales 3450, Estación Central, Santiago, Chile 6Climate and Environmental Physics, Physics Institute, University of Bern, Bern, Switzerland Correspondence to: Julien G. Anet ([email protected]) and Martin Steinbacher ([email protected]) Received: 12 July 2016 – Discussion started: 7 October 2016 Revised: 24 March 2017 – Accepted: 25 April 2017 – Published: 31 May 2017 Abstract. The knowledge of surface ozone mole fractions located over the Pacific Ocean. Therefore, due to the neg- and their global distribution is of utmost importance due to ligible influence of local processes, the ozone record also al- the impact of ozone on human health and ecosystems and the lows studying the influence of El Niño and La Niña episodes central role of ozone in controlling the oxidation capacity of on background ozone levels in South America. In agreement the troposphere. The availability of long-term ozone records with previous studies, we find that, during La Niña condi- is far better in the Northern than in the Southern Hemisphere, tions, ozone mole fractions reach higher levels than during and recent analyses of the seven accessible records in the El Niño conditions. Southern Hemisphere have shown inconclusive trends. Since late 1995, surface ozone is measured in situ at “El Tololo”, a high-altitude (2200 m a.s.l.) and pristine station in Chile (30◦ S, 71◦ W). The dataset has been recently fully quality 1 Introduction controlled and reprocessed. This study presents the observed Tropospheric ozone (O ) is a key atmospheric compound that ozone trends and annual cycles and identifies key processes 3 plays an important role in many respects: it acts as a green- driving these patterns. From 1995 to 2010, an overall pos- house gas, which is contributing to radiative forcing of up to itive trend of ∼ 0.7 ppbdecade−1 is found. Strongest trends 21 % relative to the radiative forcing induced by CO (Myhre per season are observed in March and April. Highest mole 2 et al., 2013). Ozone has adverse effects on crop yields and fractions are observed in late spring (October) and show a on human health, being an irritating agent and triggering strong correlation with ozone transported from the strato- asthma and cardiovascular diseases (Reich and Amundson, sphere down into the troposphere, as simulated with a model. 1985; Brook, 2002; Fiscus et al., 2005). Ozone is also a ma- Over the 20 years of observations, the springtime ozone max- jor source of hydroxyradicals and, thereby, influences the ox- imum has shifted to earlier times in the year, which, again, is idative capacity of the atmosphere (Crutzen, 1971; Staehelin strongly correlated with a temporal shift in the occurrence of et al., 2001). the maximum of simulated stratospheric ozone transport at Various processes determine the amount of ozone in the the site. We conclude that background ozone at El Tololo is troposphere: ozone is naturally produced by oxidation of mainly driven by stratospheric intrusions rather than photo- methane, by reaction of oxygen with lightning-induced NO chemical production from anthropogenic and biogenic pre- production, as well as by photochemical formation in the cursors. The major footprint of the sampled air masses is presence of volatile organic compounds (VOCs), nitrogen Published by Copernicus Publications on behalf of the European Geosciences Union. 6478 J. G. Anet et al.: 20 years of ozone data from El Tololo, Chile oxides (NOx), and sunlight (Crutzen, 1971, 1973; Crutzen the two hemispheres. The more pronounced trend in the NH and Zimmermann, 1991; Winer et al., 1992; Derwent et can possibly be explained by (i) higher precursor emissions al., 1998). Thus, changes in ozone precursor emissions – than in the SH and (ii) relatively short lifetime of ozone and which are partly due to anthropogenic activities – consid- subsequent lack of transport into the SH. Moreover, trends erably influence the tropospheric ozone burden. However, in the NH are very different from location to location. Re- a straightforward attribution of emission changes to ozone cent work raised the attention to the flattening of the pos- trends is challenging due to the highly nonlinear photochem- itive trend in NH tropospheric ozone at certain sites, espe- istry, different (VOC- and NOx-limited) ozone production cially at those located in Europe or eastern North America regimes, and also photochemical loss processes (Crutzen, (Cooper et al., 2014). At most stations, this finding can be ex- 1971; Sillman and He, 2002). A significant part of tro- plained by decreasing nitrogen oxides (NOx D NO C NO2) pospheric ozone originates from stratosphere–troposphere emissions in the developed western countries. Such a level- transport (STT), also known as stratosphere–troposphere ex- ing off is currently not observed in the western United States change (STE), happening, e.g., in tropopause folds (Holton as NOx sources in upstream regions such as eastern Asia are et al., 1995; Škerlak, 2014; Škerlak et al., 2014; Lefohn and still significantly increasing (Cooper et al., 2012). In contrast, Cooper, 2015). The STE is not evenly distributed over the the few SH ozone monitoring stations only partly recorded globe and hotspots of transport of stratospheric ozone into a flattening of the trend (Cooper et al., 2014). These ozone the planetary boundary layer exist in the region of the Rocky time series show either increasing positive trends (Oltmans et Mountains, Tibetan Plateau, Andes (around 30◦ S), storm al., 2013; Thompson et al., 2014) or no significant change at tracks, and Indian Ocean (Škerlak et al., 2014). Recent mod- all (Oltmans et al., 2013; Cooper et al., 2014). A worldwide eling studies postulate that the contribution from STE to the map of ozone trends interpolated from the existing surface tropospheric ozone burden may be as high as 23 % of the net ozone measurement stations is not yet available. Wespes et photochemical production (Stevenson et al., 2006; Sudo and al. (2016) recently tried to map ozone mixing ratio trends in Akimoto, 2007). This contribution may change in the future the lower troposphere by using remote sensing satellite data due to climate change and could lead to more than 20 % STE from 2008 to 2013. They showed that ozone mixing ratios increase (Collins et al., 2003; Hegglin and Shepherd, 2009; in the lower troposphere were generally decreasing all over Neu et al., 2014). the SH and in most parts of the NH during this period. How- Ozone sinks include catalytic destruction involving HO2 ever, this trend cannot be generalized as polluted areas of the and photolytic destruction; O3 can also be removed from the world still show significant positive ozone trends. atmosphere by dry deposition, wet scavenging, and uptake Tropospheric ozone records often show a pronounced sea- by vegetation (Galbally, 1968; Stevenson et al., 2006). sonal cycle. While in polluted areas a strong photochemi- The first ozone observations in the atmosphere were per- cally driven summer peak is observed, a spring peak with formed in the 19th century in Montsouris, Paris (Volz and stratospheric influence dominates in most continental pris- Kley, 1988). However, regular and geographically distributed tine regions (Wang et al., 1998a, b; Monks, 2000). Sta- measurements have become more established only in the sec- tions in the marine boundary layer in the SH such as Cape ond half of the 20th century. Nowadays, surface ozone ob- Grim, Australia, rarely reveal a distinct spring peak but rather servations are widespread and data are available from var- show a summer peak due to HO2 photochemistry (Ayers et ious data repositories such as the World Data Centre for al., 1992; Monks, 2000). Measurements in the SH free tro- Greenhouse Gases (WDCGG) of the Global Atmospheric posphere (e.g., La Quiaca, Argentina; 3459 m a.s.l.) show a Watch (GAW) programme of WMO or from regional envi- spring maximum (Barlasina et al., 2013) similar to the NH ronmental agencies like the European Environment Agency ozone time series from unpolluted stations. These findings (AirBase), the US Environmental Protection Agency (CAST- were more broadly confirmed by Cooper et al. (2014), who, NET, AQS), and the Acid Deposition Monitoring Network in using satellite-measured total column ozone datasets, clas- East Asia (EANET). Currently, observations in the Southern sified the onsets of the total column ozone (TCO) maxima Hemisphere (SH) in general, and in South America in partic- globally. In general, spring TCO maxima are found rather on ular, are very sparse (Sofen et al., 2016a, b). the SH, while summer TCO maxima are prominent in the Following Parrish et al. (2012), Oltmans et al. (2013), and NH. This latter work is in contrast to the results of Monks Cooper et al. (2014), emissions of anthropogenic volatile (2000), who concluded that the spring phenomenon is pri- organic compound and hydrocarbon emissions have led to marily a NH feature. Wang et al. (1998a) and, more recently, a strong rise of ozone production in the last century. In Lin et al. (2015) state that at least some NH spring peaks fact, ozone has been generally increasing by up to 3 and originate from a combination of ozone-rich stratospheric in- 7 ppbdecade−1 in the SH and Northern Hemisphere (NH), flux (February–April) and formation by local ozone chem- respectively, averaged over different time spans (all between istry (April–June).
Details
-
File Typepdf
-
Upload Time-
-
Content LanguagesEnglish
-
Upload UserAnonymous/Not logged-in
-
File Pages16 Page
-
File Size-