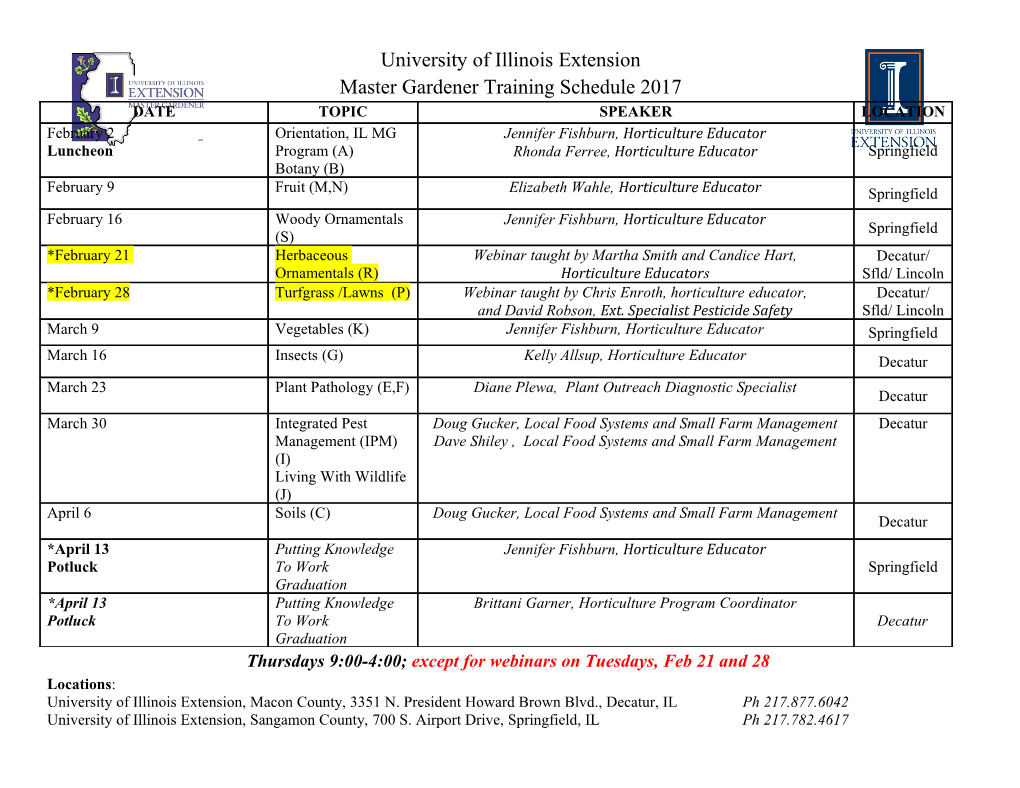
12th AIAA International Space Planes and Hypersonic Systems and Technologies AIAA 2003-7014 15 - 19 December 2003, Norfolk, Virginia HYPERSONIC AEROTHERMOELASTICITY WITH APPLICATION TO REUSABLE LAUNCH VEHICLES P.P. Friedmann,¤ J.J. McNamara,y B.J. Thuruthimattam,y and K.G. Powellz Department of Aerospace Engineering University of Michigan 3001 Fran»cois-Xavier Bagnoud Bldg., 1320 Beal Ave Ann Arbor, Michigan 48109-2140 Ph: (734) 763-2354 Fax: (734) 763-0578 email: [email protected] ABSTRACT b Semi-chord c Reference length, chord length of The hypersonic aeroelastic and aerothermoelastic double-wedge airfoil problem of a double-wedge airfoil typical cross-section CD;CL;CMy Coe±cients of drag, lift and moment is studied using three di®erent unsteady aerody- about the y-axis namic loads: (1) third-order piston theory, (2) Euler CpP T ;CpNS Piston theory pressure coe±cient, and aerodynamics, and (3) Navier-Stokes aerodynamics. CFD Navier-Stokes pressure coe±cient Computational aeroelastic response results are used respectively to obtain frequency and damping characteristics, and f(x) Function describing airfoil surface compared with those from piston theory solutions for f1(); fi() Functions relating Mach number and a variety of flight conditions. Aeroelastic behavior is temperature studied for 5 < M < 15 at altitudes of 40,000 and h Airfoil vertical displacement at Elastic 70,000 feet. A parametric study of o®sets, wedge Axis angles, and static angle of attack is conducted. All the I® Mass moment of inertia about the solutions are fairly close below the flutter boundary, Elastic Axis and di®erences between the various models increase K®;Kh Spring constants in pitch and plunge when the flutter boundary is approached. For this 2 2 respectively; K® = I®!®;Kh = m!h geometry, di®erences between viscous and inviscid L Lift per unit span aeroelastic behavior are not substantial. Results L1;L2;L3 First, second and third-order piston illustrate that aerodynamic heating reduces aeroelas- theory lift components tic stability. In addition, the hypersonic aeroelastic M free stream Mach number problem of a 3-D low aspect ratio wing, representative M; K Generalized mass and sti®ness of a ¯n or control surface present on hypersonic matrices of the structure vehicles, is studied using 1st and 3rd order piston M1;M2;M3 First, second and third-order piston theory and Euler aerodynamics. Finally, application theory moment components of this approach to a generic vehicle resembling a m Mass per unit span reusable launch vehicle is discussed. The results MEA Moment per unit span about the presented serve as a partial validation of the CFL3D Elastic Axis code for the hypersonic flight regime. Mf Flutter Mach number nm Number of modes used p Pressure NOMENCLATURE p1 Free-stream pressure Q Generalized force vector for the structure a Nondimensional o®set between the Qi Generalized force corresponding to elastic axis and the midchord mode i a1 Speed of sound q1 Dynamic pressure ¤Fran»cois-Xavier Bagnoud Professor of Aerospace Engineer- qi Modal amplitude of mode i ing, Fellow AIAA r® Nondimensional radius of gyration yPh.D. Candidate, student member AIAA S Surface area of the structure zArthur F. Thurnau Professor of Aerospace Engineering, As- S® Static mass moment of wing section sociate Fellow AIAA 1 American Institute of Aeronautics and Astronuatics Copyright © 2003 by P. Friedmann, J. McNamara, B. Thuruthimattam and K. Powell. Published by the American Institute of Aeronautics and Astronautics, Inc., with permission. about elastic axis hypersonic vehicle research program is the NASA T Temperature of airfoil, uniformly Hyper-X experimental vehicle e®ort. Other activities distributed are focused on the design of unmanned hypersonic TE Kinetic energy of the structure vehicles that meet the needs of the US Air Force. The t Time present study is aimed at enhancing the fundamental th Airfoil half thickness understanding of the aeroelastic behavior of vehicles UE Potential energy of the structure that belong to this category and operate in a typical V Free stream velocity hypersonic flight envelope. vn Normal velocity of airfoil surfaces w Displacement of the surface of the Vehicles in this category are based on a lifting structure body design. However, stringent minimum-weight x® Nondimensional o®set between the requirements imply a degree of fuselage flexibility. elastic axis and the cross-sectional Aerodynamic surfaces, needed for control, are also center of gravity flexible. Furthermore, to meet the requirement of a x; y; z Spatial Coordinates flight pro¯le that spans the Mach number range from 0 Z(x; y; t) Position of structural surface to 15, the vehicle must withstand severe aerodynamic Zeff (x) E®ective shape of double-wedge airfoil heating. These factors combine to produce unusual ® Airfoil pitch displacement about the aeroelastic problems that have received only limited Elastic Axis attention in the past. Furthermore, it is important to ®s Static angle of attack of the airfoil emphasize that testing of aeroelastically scaled wind γ Ratio of speci¯c heats tunnel models, a conventional practice in subsonic ¹m Mass ratio and supersonic flow, is not feasible in the hypersonic ½ Air density regime. Thus, the role of aeroelastic simulations is !®;!h Natural frequencies of uncoupled pitch more important for this flight regime than in any and plunge motions other flight regime. !1;!2 Natural frequencies of double-wedge airfoil Previous studies in this area can be separated into © Modal matrix several groups. The ¯rst group consists of studies fo- Ái mode shape for mode i cusing on panel flutter, which is a localized aeroelastic t ¿ Thickness ratio; ¿ = h problem representing a small portion of the skin on the b surface of the hypersonic vehicle. Hypersonic panel ³ Damping ratio flutter has been studied by a number of researchers, ()_ ; ()Ä First and second derivatives with focusing on important e®ects such as aerodynamic respect to time heating [5], composite [6, 7] and nonlinear structural () ; () Of the lower and upper surface, L U models [8], and initial panel curvature [9]. It was noted respectively in Ref. 9 that piston theory may not be appropriate for the hypersonic regime and that hypersonic studies might have to use unsteady aerodynamic loads based INTRODUCTION AND on the solution of the Navier-Stokes equations. A PROBLEM STATEMENT comprehensive review of this research can be found in a recent survey paper [10]. Hypersonic aeroelasticity and aerothermoelasticity was a vibrant and active area of research in the The second group of studies in this area was moti- late 1950's and during the 1960's as evident from vated by a previous hypersonic vehicle, namely the Refs. [1{4]. This research has been instrumental in NASP [11{17]. However, some of these studies dealt providing the basis for the aerothermoelastic design with the transonic regime, because it was perceived of the space shuttle. For a considerable time period to be quite important. Spain et al. [12] carried out a there was only limited interest in this area until the flutter analysis of all-movable NASP-like wings with advent of the National AeroSpace Plane (NASP). slab and double-wedge airfoils. They found that using e®ective shapes for the airfoils obtained by adding In recent years, renewed activity in hypersonic flight the boundary layer displacement thickness to the research has been stimulated by the need for a low airfoil thickness improved the overall agreement with cost, single-stage-to-orbit (SSTO) or two-stage-to- experiments. Aerothermoelastic analyses of NASP- orbit (TSTO) reusable launch vehicle (RLV) and the like vehicles found that aerodynamic heating altered long term design goal of incorporating air breathing the aeroelastic stability of the vehicle through the propulsion devices in this class of vehicles. The degradation of material properties and introduction X-33, an example of the former vehicle type, was a of thermal stresses [15{17]. 1/2 scale, fully functional technology demonstrator for the full scale VentureStar. Another ongoing 2 American Institute of Aeronautics and Astronuatics The third group of studies is restricted to recent papers 4. Compare the exact solutions for aeroelastic behav- that deal with the newer hypersonic con¯gurations ior using the Navier-Stokes-based unsteady air- such as the X-33 or the X-34. Reference 18 considered loads, with approximate solutions based on an air- the X-34 launch vehicle in free flight at M=8.0. foil shape modi¯ed by the presence of a boundary The aeroelastic instability of a generic hypersonic layer. vehicle, resembling the X-33, was considered in Ref. 19. It was found that at high hypersonic speeds and 5. Conduct a parametric study that illustrates the high altitudes, the hypersonic vehicle is stable, when e®ect of the o®set between the elastic axis and piston theory is used to represent the aerodynamic the aerodynamic center, the wedge angle of the loads. Sensitivity of the flutter boundaries to vehicle airfoil, and static angle of attack. flexibility and trim state were also considered [19]. In another reference [20], CFD-based flutter analysis 6. Incorporate aerodynamic heating in an approxi- was used for the aeroelastic analysis of the X-43 mate manner in order to calculate aerothermoe- con¯guration, using system identi¯cation based order lastic responses. reduction of the aerodynamic degrees of freedom. Both the structure and the fluid were discretized 7. Extend the analysis to a generic hypersonic vehi- using the ¯nite element approach. It was shown that cle that has features resembling a reusable launch piston theory and ARMA Euler calculations predicted vehicle. somewhat similar results. Finally, it is important to note that these objectives From the studies on various hypersonic vehi- not only enhance our fundamental understanding of cles [11, 20{22], one can identify operating envelopes hypersonic aeroelasticity, but also make a valuable for each vehicle.
Details
-
File Typepdf
-
Upload Time-
-
Content LanguagesEnglish
-
Upload UserAnonymous/Not logged-in
-
File Pages20 Page
-
File Size-