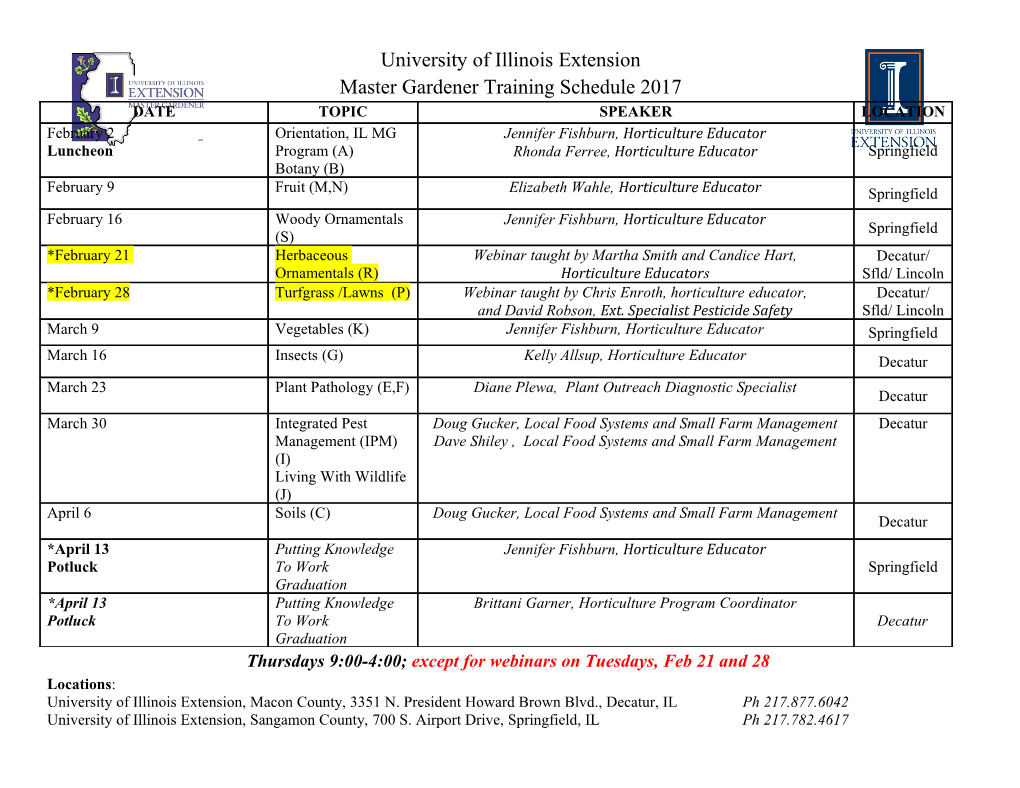
Carl Herrmann AHS Capstone Paper 3 12/17/2009 The Chemistry and Metallurgy of Iron Iron and steel workers employed a variety of techniques to convert iron ores into metallic iron, all of which utilized the same basic chemical changes. Their goal was first to liberate the metallic iron from the oxygen in iron ores, then to use oxygen to remove other impurities from the molten iron. The different techniques of doing so represented different levels of technological development, and often achieved a similar material through a less labor-intensive process. However, some methods, such as the Bessemer process, produced a different and superior material. Before the advent of cheap steel, artisanal ironworks converted iron ore into two different materials – wrought iron and cast iron. Whether producing the tough, durable wrought iron or brittle cast iron, skilled artisan converted the ore to saleable metal product with little understanding of the fundamental chemical reactions. While the chemistry behind these reactions changed little between the processes, the workers had little understanding of the chemical nature of the changes they performed, and the day to day work in forges and blast furnaces bore little resemblance to one another. Smelting iron is the conversion of mineral iron ores into metallic iron. Although their chemical composition varies according to the ores, all iron ores share one characteristic. They all contain iron and oxygen. Some, such as Hematite (Fe2O3) and Magnetite (Fe3O4), contain only these two elements, while others, such as goethite (FeO(OH)) and siderite (FeCO3 ) contain other elements. To remove the oxygen pure carbon is burned, creating CO gas. This strong reducing agent combines with the oxygen in the mineral ore, producing CO2 and leaving behind metallic iron (Gordon 90). All ores also contain other, unwanted minerals, called gangue. These are combined with a flux, often consisting of limestone, to form a slag. A slag is the term for the non-metallic compounds produced from ironmaking that chemically separate from the iron. The slag performs the useful function or removing impurities dissolved in the iron, while also acting as a chemical barrier, shielding the iron from further chemical reactions. Whether they understand the chemical processes involved, when ironworkers used different techniques to produce iron, they went about achieving these basic chemical processes in different manners, and the final material reflected these differences. Bloomeries represent the oldest and least capital-intensive method of producing iron – they required just a hearth, bellows and hammer, and were often the first method set up in a new ironworks. In a Bloomery hearth, an artisan would prepare a burning charcoal bed to provide the carbon monoxide gas to reduce the ores. Charcoal, produced by charring wood in an enclosed container, is 90% carbon (Gordon 34). While more expensive than mineral coal, it is also contains lower amounts of impurities such as phosphorus and sulfur that would affect the material properties of iron in undesirable ways; it was worth the additional expense. As it reached the proper heat, artisans spread on this bed in a particular pattern. As the ore heated up in the presence of the CO gas from the burning charcoal, chemical reactions began to take place. Silicon, a common impurity in the mineral ore, combined with some of the iron to produce Faylite (Fe2SiO4 ), a main portion of the slag (Gordon 100). This slag, common to the bloomery chemistry, contained iron and thus represents a reason why bloomery technique produces lower iron yields than other methods. As the silicon and other impurities were removed into the slag, carbon monoxide reduced the ores into metallic iron. Initially, these start as small amounts of iron which grow slowly. Although metallically bonded to the other iron atoms, other elements like carbon could diffuse into them. Carbon acted to lower the melting point of iron, reducing it so it could melt in the (relatively) moderate heat of the fire. As the iron congealed and fell through the hearth, a coating of the slag protected them from the oxygen that would convert them back into ores. When enough of these particles had congealed together, forming a ‘loup’ in the hearth, a bloomer brought the loup to a hammer. There, the white-hot metallic particles were welded together under the blows of a hammer, while the slag was squirted out. The final product of this bloomery process is known as wrought iron. The name, in fact, refers to the process of pounding it to shape it and remove the slag. This material was not a homogenous product. In fact, it was a composite mixture of relatively pure iron interspersed with solidified slag. The purity of the metallic iron made the product both highly ductile and weldable. The ductility of the material made it good for forming into various shapes, as well as strong and tough in its final application. Higher grades of wrought iron containing smaller amounts of slag could be obtained by cutting the resulting bar into strips, heating them, and once more hammering them together again. This additional hammering, often repeated several times, removed successive amounts of slag producing a progressively more pure product. While Bloomeries produced iron directly from ore, the process required a great deal of highly skilled labor and its output was limited by the size of the batches workers could handle as well as the time it took each batch to finish. While the labor required to produce iron by this method reduced by 86% over the course of the nineteenth century (Gordon 99), in the short term ironmasters interested in expanding production often invested in different techniques entirely. Similar to Bloomeries, blast furnaces use burning charcoal to produce heat and the carbon monoxide necessary to reduce iron ores to metallic iron. However, blast furnaces attain a much higher heat than bloomery hearths. While the chemical reactions taking place remain the same, the greater heat allows the products to remain molten through the process. This additional heat greatly increased the total output of the ironworks. Blast furnaces are also much larger than bloomeries, allowing them to handle much more metal (thousands of pounds of metal per batch, as opposed to hundreds). When compared to the bloomery process, they also required much less human labor and could therefore offer the final product at a lower price (Lewis 10). In contrast to bloomeries, where fine particles of iron and slag combined in a semi-solid mass shielded from further chemical reactions, in blast furnaces the liquid iron pools directly at the bottom of the hearth. The slag floats on top of this mass, preventing its oxidation. However, as the liquid iron passes through the carbon fuel, it absorbs any of the impurities present in it. These can be any from silicon to sulfur. Although usually not regarded as an impurity, the carbon absorbed into the liquid iron alters its physical properties in a significant way. The carbon present in cast iron, usually to the saturation point, results in a very strong iron. This strength, however, reduces its ductility to the point where becomes brittle. While still useful in stove plates or other applications that don’t require toughness, cast iron is useless in structural beams or most tools. It is widely used as an intermediate material in the process of refining iron, and was quite suitable in this role (Gordon 125) When cast iron intended for further processing left the blast furnace, it most often found its way to a finery, to be refined into wrought iron. Fineries worked much like bloomeries, creating a loup in a charcoal fire, and hammering the resulting iron under a large to separate the slag from the metallic iron. The final product of this was also known as wrought iron, and was indistinguishable from the bloomery product. The primary difference between the finery and the bloomery lies in the chemical reactions taking place. While the bloomery takes in ore and uses carbon monoxide to remove oxygen from the iron, a finery takes pig iron in and uses heat and oxygen to remove carbon and silicon from the cast iron. (Gordon 128). While requiring similarly skilled workers to control the chemical reactions taking place, the chemical reactions in a finery took place more quickly, so finers could produce more iron per day than bloomers. Thus by breaking down the bloomery process into two separate steps, first removing the oxygen from the iron in a blast furnace, then by removing the silicon and carbon in a finery, capitalists and managers could increase their production. However, the great skill required slowed their ability to train workers, and, because the charcoal and iron came into intimate contact, charcoal was still the only available fuel source. To address these problems, iron masters in the 1830s began using the puddling process. While similar in concept to the fining process, where pig iron is melted and exposed to air to remove excess carbon, it differs in one important aspect – the use of a reverbratory furnace in place of a hearth. A reverbratory furnace burns the fuel separately from the iron, allowing ironmasters to use cheaper mineral coal without contaminating the iron. More importantly, however, it left the molten iron in sight of the worker, making his task much easier, and therefore cheaper, to perform. (Gordon 138). In a reverbratory furnace, the coal burns on one side of a low wall; the iron sits in the hearth on the other side of the wall. On the other side of the iron sits the chimney, which draws the hot gasses over the hearth, heating the iron. In the process, the puddler inserts pig iron into the hearth.
Details
-
File Typepdf
-
Upload Time-
-
Content LanguagesEnglish
-
Upload UserAnonymous/Not logged-in
-
File Pages5 Page
-
File Size-