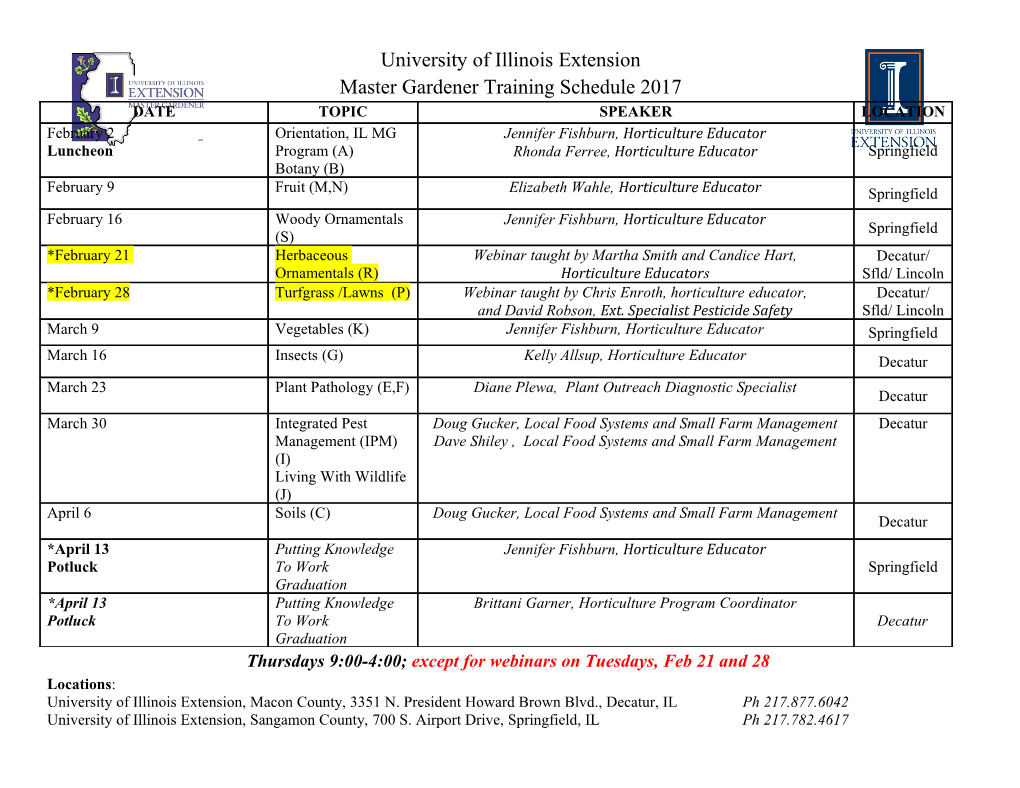
A Thesis entitled Photodissociation of (DMSO)2Fe(II)TPP, (TMSO)2Fe(II)TPP, and (PSO)2Fe(II)TPP to form a transient Five-Coordinate Complex as Studied Using Transient Resonance Raman Spectroscopy by Kenneth P. Boone Submitted in partial fulfillment of the requirements for the Master of Science Degree in Chemistry ___________________________________ Adviser: Dr. Eric W. Findsen ___________________________________ College of Graduate Studies University of Toledo December 2008 An Abstract of Photodissociation of (DMSO)2Fe(II)TPP, (TMSO)2Fe(II)TPP, and (PSO)2Fe(II)TPP to form a transient Five-Coordinate Complex as Studied Using Transient Resonance Raman Spectroscopy Kenneth P. Boone Submitted in partial fulfillment of the requirements for the Master of Science Degree in Chemistry University of Toledo December 2008 This work presents the results of UV-Visible absorption and Resonance Raman spectroscopic studies of three different heme complexes. (DMSO)2Fe(II)TPP (1) and (TMSO)2Fe(II)TPP (2) were studied in neat DMSO (dimethylsulfoxide) and neat TMSO (tetramethylene sulfoxide), respectively. A third heme complex, (PSO)2Fe(II)TPP (3), required a binary solvent of benzene and PSO (phenyl sulfoxide) in order to be created and studied. Both (1) and (2) were studied in the same binary solvent as well. The investigation determined that all three complexes behave similarly in relation to their UV-VIS and resonance Raman spectra. Resonance Raman experiments at low incident laser power have shown that (1), (2), and (3) exhibit the properties of a 6-coordinate, low- spin complex in the binary solvent. (1) and (2) exhibit the same properties in neat ii solvent. The UV-visible absorption data also provides evidence for the presence of a 6- coordinate low-spin complex for all three species in the ground state. The UV-VIS data for (2) and (3) shows a 4 and 5 nm red-shift, respectively, in their Soret bands as compared to (1) in the binary solvent. (2) displays a 3 nm red-shift in its Soret band as compared to (1) in neat solvent. These shifts are attributed to the different sulfoxide ligands bound to the iron center. For all three complexes, at high laser power, photolysis of an axial ligand occurs and a transient 5-coordinate, high-spin photoproduct is created. This transient photoproduct has a core-size of 2.05 Angstroms and 2.04 Angstroms for (1) and (2) in neat solvent. In binary solvent, the transient photoproducts for (1), (2), and (3) all possessed a calculated core-size of 2.05 Angstroms. The resonance Raman data provides evidence that the sulfoxides studied are moderate σ−donors and π−acceptors when compared to nitrogenous ligands such as imidazole and pyridine. However, there is no evidence in the resonance Raman data to distinguish among the three sulfoxides the strongest π-acceptor. iii Acknowledgements I would like to first of all thank my research director, Dr. Eric W. Findsen, for his training in the lab, advice, and for taking me out to dine at Taco Bell and Ferdo’s. I would also like to thank the members of my committee Dr. Mark Mason and Dr. Xiche Hu for their assistance. I would also like to thank former graduate students Eric Yearley and Aaron Duckworth for listening when I had to vent. Aaron’s family was very helpful in allowing me to stay at their house two to three times per week. They welcomed me into their house and I will never forget them or the kindness they displayed. I cannot forget the secretaries Charlene Hansen and Pam Samples, they were life-savers when help was needed to navigate the maze of paperwork associated with working in the Chemistry Department of a large university. Last, and certaintly not least, I would like to thank my wife Sarah for her love and support. I could not have survived graduate school without her help. Sincerely, Kenneth Patrick Boone iv Table of Contents Abstract ii Acknowledgements iv Table of Contents v List of Figures vi List of Tables vii I. Introduction 1 II. Experimental Methods 6 III. Theory 13 IV. UV-Visible Absorption Spectroscopy of Ferrous Tetraphenylporphyrin Complexes 33 V. Nanosecond Transient Resonance Raman Spectroscopy of Tetraphenylporphyrin Complexes 38 VI. Discussion and Conclusions 50 VII. Bibliography 58 v List of Figures Figure 2.1 Schematic diagram for Raman Instrument. 11 Figure 2.2 Picture of (PSO)2Fe(II)TPP solution. 12 Figure 3.1 Structure of a metalloporphyrin ring 14 Figure 3.2 Porphyrin frontier molecular orbitals. 16 Figure 3.3 Schematic of Gouterman’s Four-Orbital Model. 17 Figure 3.4 Schematic for the d-orbital energy splittings for ferrous porphyrins. 20 Figure 4.1 UV-vis absorption spectra of A) (DMSO)2Fe(II)TPP and B) (TMSO)2Fe(II)TPP in neat solvent. 36 Figure 4.2 UV-vis absorption spectra of A) (DMSO)2Fe(II)TPP, B) (TMSO)2Fe(II)TPP, and C) (PSO)2Fe(II)TPP in the binary solvent. 37 Figure 5.1 10-ns resonance Raman spectra of (DMSO)2Fe(II)TPP in neat solvent (high and low power). 45 Figure 5.2 10-ns resonance Raman spectra of (TMSO)2Fe(II)TPP in neat solvent (high and low power). 46 Figure 5.3 10-ns resonance Raman spectra of (PSO)2Fe(II)TPP in binary solvent (high and low power). 47 Figure 5.4 10-ns resonance Raman spectra of (TMSO)2Fe(II)TPP in binary solvent (high and low power). 48 Figure 5.5 10-ns resonance Raman spectra of (DMSO)2Fe(II)TPP in binary solvent (high and low power). 49 Figure 6.1 Overall dynamics scheme for the porphyrin systems investigated. 55 vi List of Tables Table 2.1 Abbreviations for ligands and porphyrins used in this investigation. 8 Table 3.1 Observed center-to-nitrogen distances for ferrous porphyrins obtained from crystallographic data. 22 Table 3.2 Expected spin-states and structures for ferrous porphyrins. 22 Table 4.1 UV-visible absorption band wavelengths for six- and five- coordinate ferrous porphyrin complexes. 35 Table 5.1 Comparison of experimentally determined resonance Raman frequencies for ferrous iron porphyrin complexes containing either DMSO, TMSO, or PSO. 43 Table 5.2 Resonance Raman frequencies for various five- and six-coordinate complexes. 44 Table 5.3 Calculated ferrous tetraphenylporphyrin center to nitrogen (Ct-N) distances. 44 vii Chapter 1 Introduction Tetraphenylporphyrins belong to the broad class of porphyrins referred to as synthetic. Some synthetic porphyrins provide ideal four-fold symmetry and while alterations to the periphery of physiological-type porphyrins produce subtle changes in the vibrational spectrum, these synthetic porphyrins present a substantially altered vibrational pattern that is still recognizable. Although natural porphyrins should serve as the best models for proteins, there are advantages in studying the synthetic porphyrins in many circumstances. One advantage of using meso-aryl substituted porphyrins is that they tend to aggregate much less1 than do natural porphyrins.2 Second, in many cases, the low symmetry of natural porphyrins limits the spectral resolution of the vibrational modes. As will be seen from the vibrational spectra, the increase in symmetry increases the resolution of the symmetrical vibrational bands of the porphyrin. A third advantage is that these porphyrins are easy to prepare and to crystallize, which has made them very useful in structural studies.3,4 The focus of this study is on three model tetraphenylporphyrin complexes with the iron in the +2 oxidation state. This allows direct comparison to be made to model heme systems (such as iron (II) protoporphyrin IX) and to hemeproteins such as oxyhemoglobin, myoglobin, and intermediate complexes of catalase and cytochromes. 1 Model porphyrin systems of interest are those with one or more axial ligands which either mimic or contrast to those in the active sites of hemeproteins. Sulfoxide ligands, such as DMSO (dimethyl sulfoxide) and TMSO (tetramethylene sulfoxide) have been utilized as solvents for electrochemical applications and in ligand binding studies to iron porphyrins.5,6 However, when compared to oxygen, carbon monoxide, and nitrogen-based ligands, sulfoxide ligands have been relatively ignored. Only a few investigations have been carried out where at least one of the ligands on the ferrous model porphyrin has been a sulfoxide ligand.7,8 In this study, several sulfoxides were used as axial ligands to study metalloporphyrin macrocycle dynamics. Investigation of model metalloporphyrin complexes with two sulfoxide ligands is important for several reasons. Most of the model metalloporphyrin complexes that have been characterized have at least one nitrogenous-base axial ligand. Investigation into other types of ligands clarifies properties of the complex which are due to nitrogenous-base ligands such as imidazole. The DMSO ligand (which has been shown to bind through the oxygen atom3,9 may be a 10 good model for catalase and oxygen intermediates of cytochrome P450. The excited state dynamics of these model metalloporphyrin systems are useful in understanding the excited-state behavior of heme active sites and in potential applications of iron porphyrin complexes in photochemical systems. Ligand photodissociation dynamics and the excited state decay mechanisms of other model iron (II) porphyrin complexes and hemeproteins have been studied. The primary tool for the investigations, however, has been transient absorption spectroscopy. The time-resolved studies have been carried out on model iron (II) porphyrins with 2 ligands such as nitrogenous bases,12 carbon monoxide/mixed ligand complexes,13,14,15 isocyanides,16 and nitrosyl ligands.17 Efforts have been made to detect and characterize the electronic excited state intermediates of hemes and hemeproteins by transient absorption spectroscopy, but the number of excited-state intermediates and their timescales are in dispute.40 Resonance Raman spectroscopy has been used to study model heme systems but those investigations have focused on the equilibrium structure of the porphyrin.
Details
-
File Typepdf
-
Upload Time-
-
Content LanguagesEnglish
-
Upload UserAnonymous/Not logged-in
-
File Pages69 Page
-
File Size-