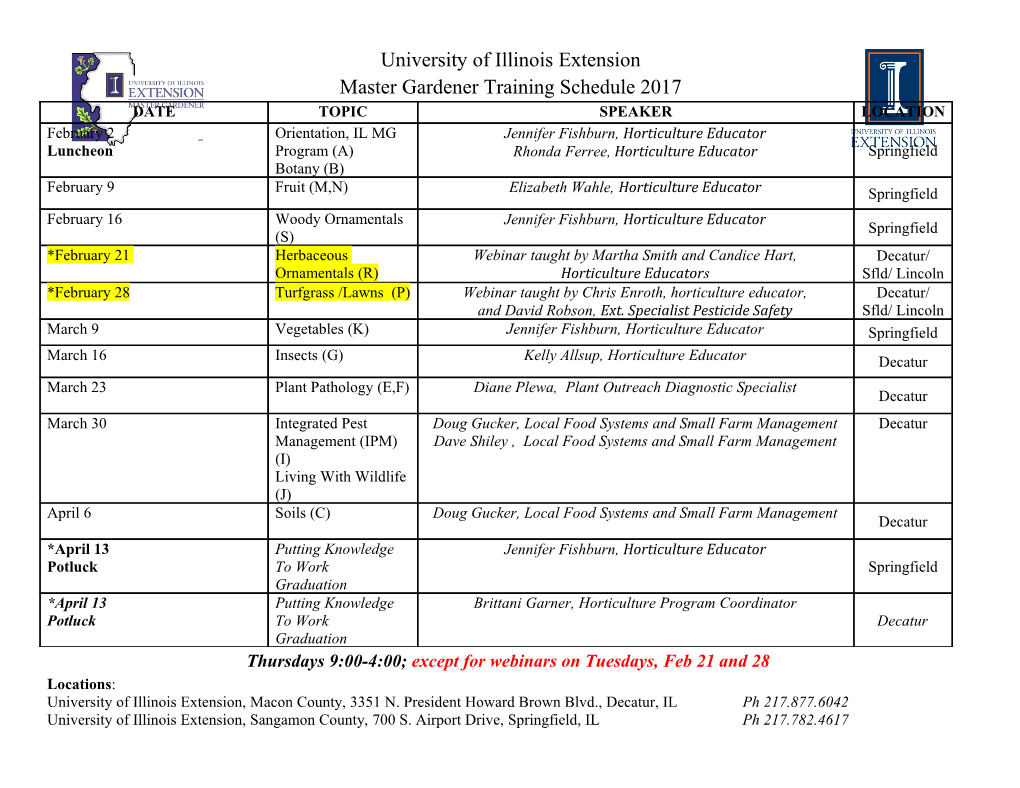
University of Southampton Research Repository ePrints Soton Copyright © and Moral Rights for this thesis are retained by the author and/or other copyright owners. A copy can be downloaded for personal non-commercial research or study, without prior permission or charge. This thesis cannot be reproduced or quoted extensively from without first obtaining permission in writing from the copyright holder/s. The content must not be changed in any way or sold commercially in any format or medium without the formal permission of the copyright holders. When referring to this work, full bibliographic details including the author, title, awarding institution and date of the thesis must be given e.g. AUTHOR (year of submission) "Full thesis title", University of Southampton, name of the University School or Department, PhD Thesis, pagination http://eprints.soton.ac.uk UNIVERSITY OF SOUTHAMPTON FACULTY OF PHYSICAL AND APPLIED SCIENCES Electronics and Computer Science Development and Implementation of a Deflection Amplification Mechanism for Capacitive Accelerometers by Ioannis Zeimpekis A Thesis for the degree of Doctor of Philosophy January 2012 UNIVERSITY OF SOUTHAMPTON ABSTRACT FACULTY OF PHYSICAL AND APPLIED SCIENCES ELECTRONICS AND COMPUTER SCIENCE Doctor of Philosophy Ioannis Zeimpekis Micro-Electro-Mechanical-Systems (MEMS) and especially physical sensors are part of a flourishing market ranging from consumer electronics to space applications. They have seen a great evolution throughout the last decades, and there is still considerable research effort for further improving their performance. This is reflected by the plethora of commercial applications using them but also by the demand from industry for better specifications. This demand together with the needs of novel applications fuels the research for better physical sensors. Applications such as inertial, seismic, and precision tilt sensing demand very high sensitivity and low noise. Bulk micromachined capacitive inertial sensors seem to be the most viable solution as they offer a large inertial mass, high sensitivity, good noise performance, they are easy to interface with, and of low cost. The aim of this thesis is to improve the performance of bulk micromachined capacitive sensors by enhancing their sensitivity and noise floor. MEMS physical sensors, most commonly, rely on force coupling and a resulting deflection of a proof mass or membrane to produce an output proportional to a stimulus of the physical quantity to be measured. Therefore, the sensitivity to a physical quantity may be improved by increasing the resulting deflection of a sensor. The work presented in this thesis introduces an approach based on a mechanical motion amplifier with the potential to improve the performance of mechanical MEMS sensors that rely on deflection to produce an output signal. The mechanical amplifier is integrated with the suspension system of a sensor. It comprises a system of micromachined levers (microlevers) to enhance the deflection of iii iv Abstract a proof mass caused by an inertial force. The mechanism can be used in capacitive accelerometers and gyroscopes to improve their performance by increasing their output signal. As the noise contribution of the electronic read-out circuit of a MEMS sensor is, to first order, independent of the amplitude of its input signal, the overall signal-to- noise ratio (SNR) of the sensor is improved. There is a rather limited number of reports in the literature for mechanical amplification in MEMS devices, especially when applied to amplify the deflection of inertial sensors. In this study, after a literature review, mathematical and computational methods to analyse the behaviour of microlevers were considered. By using these methods the mechanical and geometrical characteristics of microlevers components were evaluated. In order to prove the concept, a system of microlevers was implemented as a mechanical amplifier in capacitive accelerometers. All the mechanical structures were simulated using Finite Element Analysis (FEA) and system level simulations. This led to first order optimised devices that were used to design appropriate masks for fabrication. Two main fabrication processes were used; a Silicon on Insulator (SOI) process and a Silicon on Glass (SoG) process. The SOI process carried out at the University of Southampton evolved from a one mask to a two mask dicing free process with a yield of over 95%, in its third generation. The SoG is a well-established process at the University of Peking that uses three masks. The sensors were evaluated using both optical and electrical means. The results from the first prototype sensor design (1HAN) revealed an amplification factor of 40 and a mechanically amplified sensitivity of 2.39V/g. The measured natural frequency of the first mode of the sensor was at 734Hz and the full-scale measurement range was up to 7g with a maximum nonlinearity of 2%. The measurements for all the prototype sensor designs were very close to the predicted values with the highest discrepancy being 22%. The results of this research show that mechanical amplification is a very promising concept that can offer increased sensitivity in inertial sensors without increasing the noise. Experimental results show that there is plenty of room for improvement and that viable solutions may be produced by using the presented approach. The applications of this scheme are not restricted only to inertial sensors but as the results show it can be used in a broader range of micromachined devices. Contents Contents .............................................................................................................. v List of figures ............................................................................................................. ix List of tables ........................................................................................................... xxi List of abbreviations ................................................................................................. xxiii Declaration of authorship ......................................................................................... xxv Acknowledgements .................................................................................................. xxvii Chapter 1 Introduction ......................................................................................... 1 1.1 Overview of research ..................................................................................... 1 1.2 Motivation and contributions ......................................................................... 2 1.3 Document structure ........................................................................................ 4 Chapter 2 Literature review ................................................................................ 7 2.1 Introduction to mechanical amplification ...................................................... 7 2.2 Introduction to compliant mechanisms .......................................................... 8 2.3 Flexure hinges .............................................................................................. 10 2.4 Mechanical amplification mechanisms in MEMS devices .......................... 12 2.4.1 Piezoelectric micropositioning stage with high resolution and amplitude ......................................................................................................... 12 2.4.2 Electrostatic parallelogram actuators ............................................... 15 2.4.3 Micromotion amplifier ..................................................................... 17 2.4.4 Two-axis scanner array driven by a force amplifying leverage mechanism ......................................................................................................... 19 2.4.5 Bridge type flexure mechanism ....................................................... 21 2.5 Mechanical amplification in inertial sensors ............................................... 24 2.5.1 Non-resonant micromachined gyroscope with structural mode decoupling ......................................................................................................... 24 2.5.2 Mechanical amplification in MEMS devices based on topology, shape and size optimisation ................................................................................... 25 2.5.3 Topology optimisation of a force amplifier on a resonant accelerometer ......................................................................................................... 29 2.5.4 Single stage amplification in a resonant accelerometer ................... 30 v vi Contents 2.5.5 Dual stage mechanical amplification ............................................... 33 2.6 Conclusions on the literature review ............................................................ 34 Chapter 3 Microlevers ........................................................................................ 37 3.1 Introduction .................................................................................................. 37 3.2 Types of microlevers .................................................................................... 37 3.3 Microlever model analysis ........................................................................... 39 3.3.1 Pseudo rigid body model .................................................................. 40 3.3.2 Static analysis using a model based on stiffness, deflections and deformations .........................................................................................................
Details
-
File Typepdf
-
Upload Time-
-
Content LanguagesEnglish
-
Upload UserAnonymous/Not logged-in
-
File Pages227 Page
-
File Size-