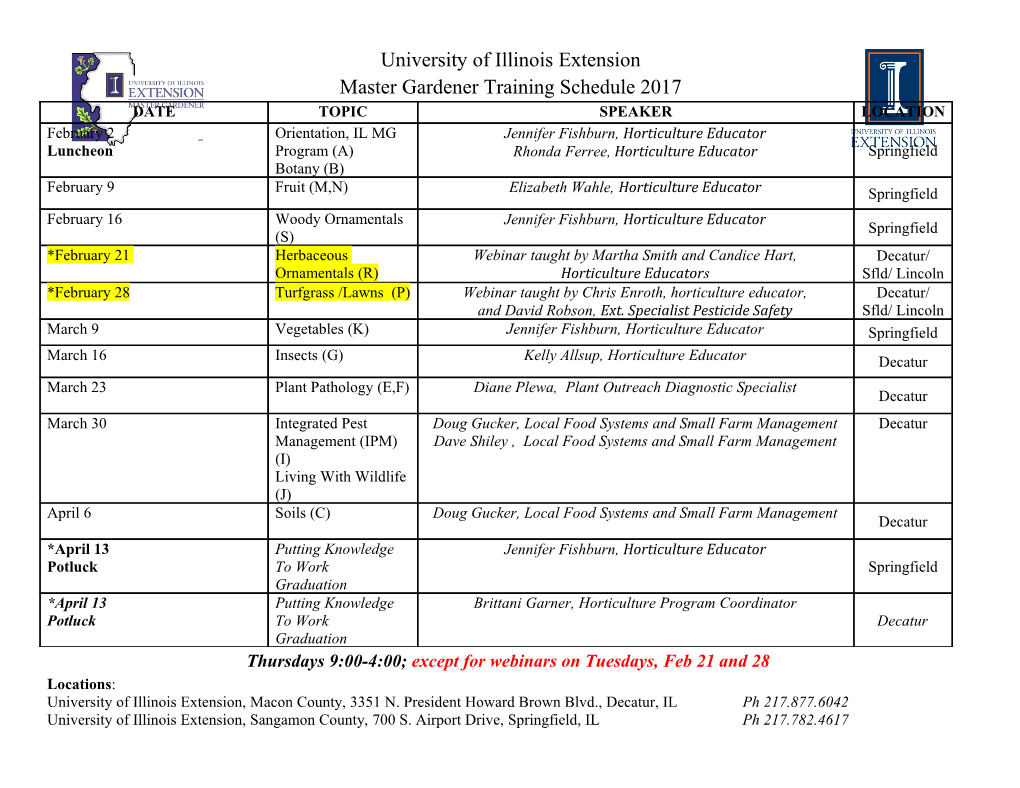
Backgrounds and Detector Issues at a Muon Collider S. Geer Fermi National Accelerator Laboratory, P.O. Box 500, Batavia, Il 60510 ABSTRACT environment (maybe it can't !). This second problem was con- sidered in Refs. [3, 4], was subsequently the subject of a working Backgrounds arising from muon decay at a 4 TeV muon col- sub-group [5] at Snowmass, and is the subject of this paper. lider are summarized, and some implicationsfor a muon collider In the following we consider a 2+2 TeV muon collider with detector are discussed. Ideas on how to cope with the signi®cant 12 10 two bunches of 2 muons per bunch, a luminosity of background levels are also described. 35 2 1 ? =3 10 cm s , mm, and a beam-beam interaction re- gion 3 mm long and 3 m radial rms. The time between bunch- I. INTRODUCTION bunch crossings is about 10 s. In Section II a summary of our current understanding of the background ¯uxes is presented. In The physics case for a multi-TeV lepton collider has been ex- Section III some general detector considerations are discussed. tensively studied [1]. It is generally believed that new physics Sections IV and V discuss the background implications for ver- associated with electroweak symmetry breaking will manifest it- tex and outer tracking detectors, together with some ideas for self at or below the few TeV scale. If this new physics gives rise trackers that have been discussed at Snowmass and are worthy of to new particles (e.g. SUSY,technicolor, extended gauge groups further consideration. Sections VI and VII discuss electromag- with new gauge bosons, ...) precise measurements of their prop- netic and hadronic calorimeter performance, and Section VIII erties will be essential to obtain a full understanding of the un- discusses muon detection. Finally, a summary is given in Sec- derlying physics. A lepton collider would seem to be the tool of tion IX. choice for these precision measurements. A multi-TeV lepton collider will be needed if any of the new particles have masses close to 1 TeV or above, or if no new particles are discovered II. BACKGROUNDS below 1 TeV in which case precise measurements of longitu- The main backgrounds at a muon collider are expected to dinal WW scattering at high-energy are important. Hopefully come from the interactions of high energy electrons produced we will begin to explore some of this new physics at TEV33, 12 10 by muon decay. With 2 muons per bunch and a beam LEP2, and/or the LHC. However, it seems likely that our knowl- 5 10 energy of 2 TeV there will be 2 muon decays per meter edge of any new physics beyond the Standard Model obtained producing electrons with a mean energy of 700 GeV (see Fig. 1). at these machines will be incomplete, and that either a multi- These electrons are emitted at very small angles with respect to TeV lepton collider or a very highenergy hadron collider willbe- the beam direction, and hence stay within the beam-envelope come essential to move beyond the LHC energy scale. Unfortu- + until they see the magnetic ®elds of, for example, the ®nal fo- e nately the performance of a multi-TeV e collider is severely cus quadrupoles. On average, as the decay electrons traverse limited by beamstrahlung and (for circular machines) by syn- the ®elds of the ®nal focus system they radiate 300 synchrotron chrotron radiation. Furthermore, the two full energy linacs re- photons with a mean energy of about 500 MeV, and therefore + e quired for a linear e collider may not be affordable. A pos- loose on average 20% of their energy before being swept out of sible solution is to build a muon collider. Since the muon is 207 the beam-pipe. The electrons can then interact in the beam-pipe times heavier than the electron, beamstrahlung is not a severe walls, beam elements, or shielding to initiate electromagnetic problem [2] and the drastic reduction in synchrotron radiation showers. Important secondary interactions that contribute to the permits a circular collider. In addition, a multi-TeV muon col- overall background ¯ux are Bethe-Heitler muon pair production lider would have the added bonuses that (i) the reduced beam- + !Z in the ®elds of the atomic nucleii ( Z ), muon pair strahlung results in a reduced spread in center-of-mass energy + + e ! production by electron±positron annihilation (e ), yielding more precise energy scans, and (ii) for s-channel cross- and photonuclear interactions that result in a large ¯ux of low en- sections that grow with mass squared (e.g. Higgs production) a 2 ergy protons and neutrons, and produce additional muons from muon collider has an advantage of (207) over an electron col- hadron decay. The result of all these electron-induced interac- lider. If this was the whole story then the muon collider would tions is a large ¯ux of low energy electrons, photons, charged be an obvious choice for a multi-TeV lepton collider. However, hadrons, and neutrons that are incident upon the detector volume there are two major problems that must be overcome. First, more together with a signi®cant ¯ux of higher energy prompt muons work is needed before it can be demonstrated that a muon col- almost parallel to the beam directions. A careful design of the lider will work (maybe it won't !). Second, muons decay giving ®nal focus system and the shielding immediately before the de- rise to a large background ¯ux through the detector. It has yet tector can reduce this background by several orders of magni- to be demonstrated that physics can be done in this background tude [4]. Obtaining the optimal con®guration is an iterative pro- Work supported by the U.S. Dept. of Energy under contract DE-AC02- cess which has not yet been completed. However, a factor of 76CH03000 100 reduction in the predicted background ¯ux has already been 453 QUAD Polyboron Fe SHIELDING CONFIGURATION W Cu 10 cm W 4 mrad IP 110 cm Figure 1: Energy distributionof electrons from 50000 simulated 6.5 m decays of 2 TeV muons. Courtesy of T. Diehl. Figure 3: Shielding con®guration implemented in the GEANT background calculation. obtained. Furthermore, several further improvements to the lat- tice and shielding have been discussed at Snowmass and are ex- A. Background Calculations pected to lead to an additional reduction of the predicted back- grounds. A correct understanding of the backgrounds is essential in or- The present background calculations, which provide a de- der to develop a reasonable strawman detector design and under- tailed simulation of all of the effects listed above, are described stand the feasibility of doing physics at a muon collider. Two in- in Ref. [6] and summarized in the following sub-sections. Beam dependent detailed background simulation programs have been halo and beam-beam interactions will also contribute to the developed. The ®rst calculation has been developed by I. Stumer backgrounds seen by the detector. There will need to be a very and is based on version 3.21 of the GEANT code used to- ef®cient scraping system to eliminate beam halo on the far-side gether with EGS [7] for electromagnetic shower simulation, of the collider ring. This system has not yet been designed, and FLUKA [8] to propogate hadronic showers, and MICAP [9] to a model for the halo has not yet been developed. Hence beam- transport low energy neutrons. The second calculation has been halo backgrounds are not included in the present calculations. developed by N. Mokhov and is based on the MARS code [10]. The beam-beam interaction is also not yet in the background These calculations have used different lattices, different shield- simulation. Eventually it will have to be included, however it ing con®gurations, and different particle dependent energy cut- is believed that backgrounds from this source will be relatively offs. In general the results from the two calculations are simi- small [2]. lar. Where there are signi®cant differences, they can be under- stood in terms of the differences in the details implemented in the calculations. In the following the ®nal focus and shielding con®guration used in the GEANT calculation is described. The exp. hall final focus corresponding details for the MARS calculation can be found in Ref. [4]. The ®nal focus geometry implemented in the GEANT calcu- Q66 Q55 Q44 Q33 lation is shown in Fig. 2. The straight section before the inter- action point (IP) is 130 m long, and consists of an 80 m long re- gion containing no magnets followed by a 50 m long ®nal fo- T56 T45 T34 cus region which accommodates the 4 ®nal focus quadrupoles, 3 toroids, and an experimental hall containing the detector plus shielding. The toroids ful®ll a double role; ®rstly they are scrap- ers for the electromagnetic debris, and secondly they sweep Figure 2: Region around the IP modelled in GEANT. The ex- prompt muons away from the detector. The last 6.5 m before perimental hall and the ®nal 50 m of the straight section imme- the IP is used for shielding to reduce the backgrounds in the de- diately before the IP are shown. tector volume as much as possible. The shielding occupies two cones that point at the IP with cone angles of 20 . The shielding 454 105 104 n 3 γ 10 102 101 e ± 0 ± 10 h 10-1 µ 10-2 10-3 Particle Fluence (per cm**2 per X-sing) 10-4 SVD Tracker ECAL HCAL 10-5 0 50 100 150 200 Radius (cm) Figure 4: GEANT results: Radial particle ¯uxes shown as a Figure 5: MARS results: Particle ¯uences (track length per unit function of radius in the detector volume.
Details
-
File Typepdf
-
Upload Time-
-
Content LanguagesEnglish
-
Upload UserAnonymous/Not logged-in
-
File Pages10 Page
-
File Size-