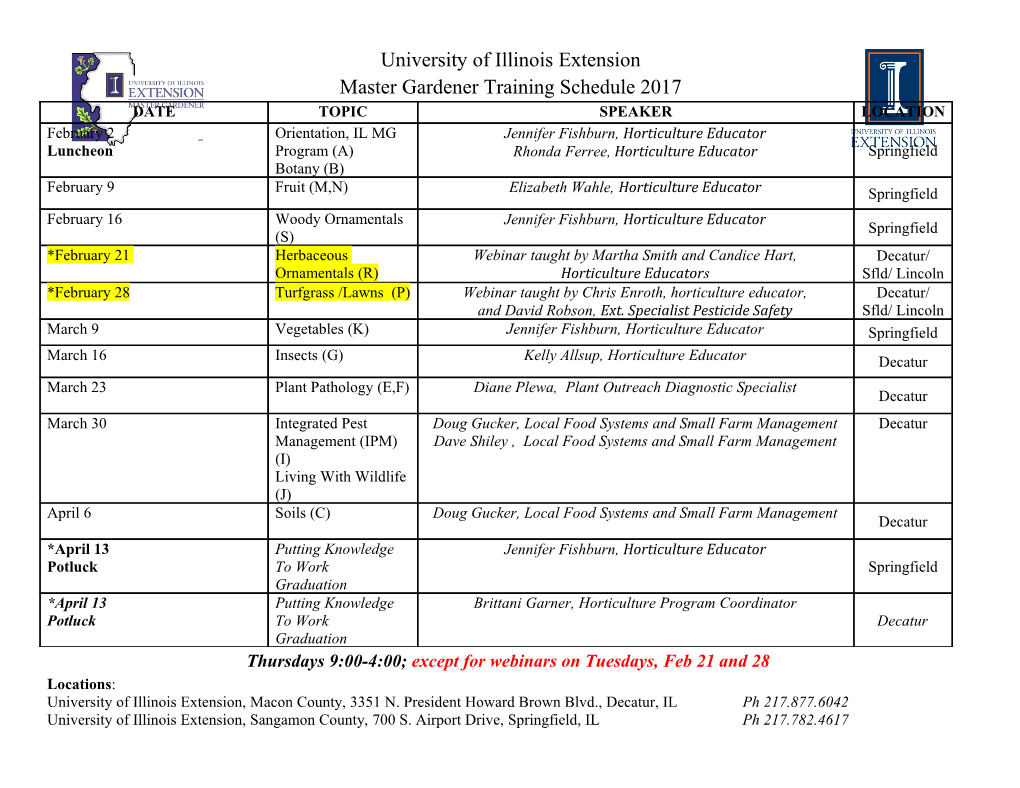
Jung-Yong Lee1, Steve T. Connor2, Ragip Pala3, Edward Barnard3, Shigeo Fujimori1,Yi Cui3, Mark L. Brongersma 3, Shanhui Fan1, and Peter Peumans1 1Department of Electrical Engineering, Stanford University Plasmonic Solar Cells 2Department of Chemistry, Stanford University 3Department of Materials Science and Engineering, Stanford University Introduction Localized Fields Deposition Technology Metal Gratings and Nanowires Electrodes Overall Quantum Efficiency Deposition Technology for Fundamental problem: L <<1/α Localized Photon Absorption Metal Gratings in Solar Cells Silver Nanowires Film D Organic/Inorganic Composite Systems ~LA Metal grating 20 ηED ~2xL MTDATA 15nm D 16 multilayer y (1) ηA > 50% CuPc 30nm deposition •Conventional transparent nc 12 to pump to pump PTCBI 30nm electrodes such as Indium- 8 que BCP 15nm e (2) ηED ~ 10% mixing with Tin-Oxide (ITO) are 4 Fr substrate organic problematic: Metal electrode 0 heater molecules Substrate 048121620 •Expensive (3) ηCT ~ 100% substrate 500nm Nanowire Length (μm) •Brittle (crack when bent) pyrex 20 sleeve •Resistive OR transparent (4) η ~ 100% nanoparticle •Cannot be deposited on 15 CC hν hν aerosol 10μm top of organic 500nm 10 Exciton diffusion length ~ 5-20 nm Finite Element Method 1.0 1600 5 •Metals are cheap (e.g. Ag) TE 0 Nanoparticle: Ag (radius = 5nm) 60 80 100 120 140 160 Carrier gas evaporate in thin film form and very TM η = η . η . η . η ~ 10% Organic: copper phthalocyanine (CuPc) (N2, Ar, …) solvent 0.8 Nanowire Diameter (nm) EQE A ED CT CC ultrasonic conductive but opaque m) μ Wavelength: 450nm / atomizer 2 e m / 2 •Can we nanostructure nc 0.6 Maximum exciton generation rate ∝ |E| : ~70 times enhancement atomized a t t suspension i 3.5e − 20 organic sources metals such that they 800 e (W Organic Photovoltaic Cell with Ag NWs ≅ 5 m Calculated absorbed power enhancement: times Carrier gas s Plasmonic Solar Cell 7.0e − 21 (N2, Ar, …) become optically 0.4 1.0 an dianc r a transparent without losing T r Simple model system: Ag cylinders in DA solar cell r 40nm I conductivity? 0.8 R =10.3Ω Model: optical absorption + exciton diffusion Broadband Resonance: Increased Why Use Vapor Phase? 0.2 400nm 0.95 sh R =22.1Ω 100nm y sh Exciton quenching at Ag interface not modeled t Solar 0.90 vi Absorption Near and Off Resonance 0.6 i • Materials use efficiency >50% iss ance 0.85 Ag nano-cylinders 0.0 0 t -19 -19 sm x 10 x 10 400 600 800 1000 • Multilayers n a 0.80 w/ nanoparticle w/ nanoparticle 0.4 r CuPc PTCBI CuPc • Additional degrees of freedom in controlling nanostructure (pressure, Wavelength (nm) T r w/o nanoparticle w/o nanoparticle ansmit la 0.75 r temperature, gas flow) o S PTCBI T 0.2 0.70 Shtein, Peumans, Benziger and Forrest, 0.00.10.20.30.40.50.6 2 2 • Rates as high as 1μm/s Adv. Mater. 16, p.1615 (2004). λ=600nm λ=600nm -2 Ag cylinders cooled baffles Nanowire Density (μm ) ] . 0.0 s 400 500 600 700 800 b cooled boundary to pump substrate layer Wavelength (nm) 1 1 300K 300K TM TE 300K B E light dark ent [ar no metal E B 2 r light Ag NWs mesh electrode ur Absorbed power Absorbed Power ITO electrode ] 2 0 0 m 1 900K 300 400 500 600 700 800 900 300 400 500 600 700 800 900 900K Photoc •Calculation of: Wavelength (nm) A/c Air cylinders Wavelength (nm) heated chamber wall chamber heated wall chamber heated •optical energy flow (streamline) 0 •optical electric field (colormap) t [m en •Substantial increase in optical absorption near plasmon •Metal gratings are excellent transparent contacts to solar cells r 473K -1 300 400 500 600 700 800 900 resonance of the Ag nanoparticles Cur source barrel source Wavelength [nm] filler flow •Even off-resonance: scattered field enhance absorption source hot Metal Gratings and Nanowires mesh are -2 cold source cold source flows -0.5 0.0 0.5 Mechanisms (a) Carrier gas flow (b) Temperature (c) Concentration (d) Molecular flow Reduced PL Quenching Very Good Transparent Contacts Voltage (V) 1.0 Localized photon absorption near the DA interface: •Ag/SiO2 particles embedded in organic thin films enhance absorption •SiO shell is essential (bare Ag particles leads to PL quenching) Thin Film by Aerosol Deposition metal grating •Increase optical electric field near the DA 2 0.9 5 interface within an exciton diffusion length x 10 14 y t i Conclusions • Can deposit films at very low solubility (e.g. 0.0001wt%) w/o Ag v 0.8 glass Absorbed Power i Exciton Density w/ Ag+SiO s 2 Density TEM Image • 10nm roughness for 100nm films is 12 w/ bare Ag m 0.7 s ITO ] . an s glass b With Ag/SiO2 nanoparticles Terrylene on Si Tr 0.6 10 lar CNT/metal NW mesh o Ag gratings (theory) •More excitons can be created by e [ar No Ag nanoparticles S 0.5 ITO (modeled) 8 enc Ag core CNT mesh plasmonic effects using metal nanoparticles c on glass i s 0.4 Ag NW mesh s i SiO shell em 2 ines 6 10 100 Sheet Resistance (Ω/sq) •Insulator shell prevents exciton quenching |E| n(x,y) 4 Photolum •Metal nanoparticles may accelerate •Increased exciton diffusion length With Ag nanoparticles •Transmittance depends on geometrical factors, such as grating period, 2 aperture ratio, thickness, etc. exciton diffusion •For both TM and TE polarizations, transmittances is better than that of ITO for comparable sheet resistance 0 400 450 500 550 600 650 •Metal core/insulator shell particles are Wavelength [nm] interesting additives to organic solar cells Growth of Metal Nanowires •Metal gratings with appropriate period and Enhanced Exciton Diffusion duty rate, etc. can be more transparent than 1. Template-Assisted Electroplating Enhanced exciton diffusion Monte Carlo Simulations 2. Shape-Controlled Synthesis of Metal conventional transparent electrodes. Template Assisted Electroplating provides required fine control 1. Start at (0, 0, 0). Nanoparticles •Exciton = oscillating dipole. dQ 1 2 • Fine control of wire length '' 2. Calculate the magnitude of electric field, |E| at each 1 nm lattice grid. Small diameters possible (10nm) •New device performance can be as good R j = = ωε E j • •Power absorption at point R: 3. Rate to transfer to grid j is, 2 3mL 0.1M AgNO injected into 5mL dt 2 R ∝ E • Multiple metals in the same wire 3 as conventional organic PV cells. j 0.3M PVP in ethylene glycol at 170ºC R j 4. The probability to transfer to the grid j is, Porous template in 20 aliquots. •Transfer probability: Pj = , (i = lattice number) R j •Metal nanowires are candidates for the Ri ∑ Pj = Ag nanosphere Ri r=5nm, d =3nm, spacing=24nm ∑ replacement of IT O. coating electrode layer 0.9 exciton With Ag r 0.8 •Organic Photovoltaic cells with Ag NWs e Without Ag 1µm l 1µm sf 0.7 Anodized Alumina Templates el Virtual shell n mesh are demonstrated and shows a 0.6 sh Available Pore Diameters: 10 nm - 1 µm tr o m 0.5 comparable performance to the t y 1n 0.4 ilit n conventional cells. i 1nm b h 0.3 a t Synkera Technologies, Inc i b w o 0.2 The authors gratefully acknowledge financial Pr 0.1 0 M. Barbic & A. Scherer, Nano Let. 2005 support from GCEP and NSF. exciton 1 2 3 4 5 6 7 8 9 10 Distance from the exciton (nm) Average step size: 1.3 nm Average step size: 2.8 nm.
Details
-
File Typepdf
-
Upload Time-
-
Content LanguagesEnglish
-
Upload UserAnonymous/Not logged-in
-
File Pages1 Page
-
File Size-