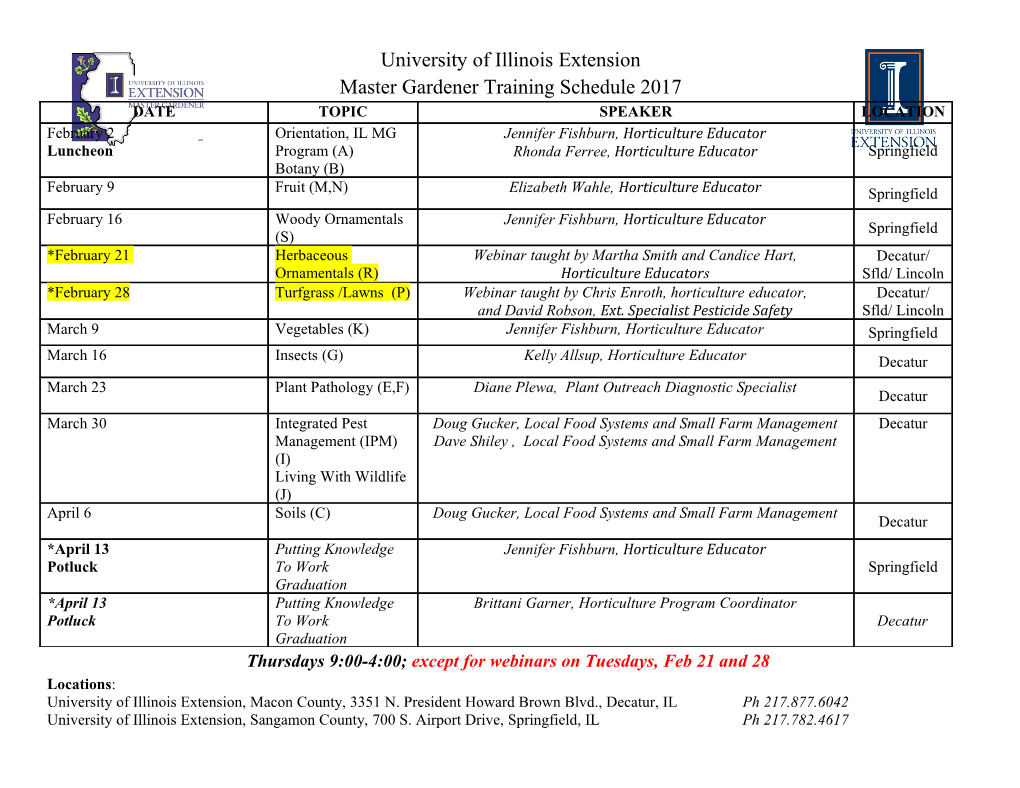
Background Results Sketch of Proof The Explicit Sato-Tate Conjecture in Arithmetic Progressions Trajan Hammonds, Casimir Kothari, Hunter Wieman [email protected], [email protected], [email protected] Joint with Noah Luntzlara, Steven J. Miller, and Jesse Thorner October 7, 2018, Qu´ebec-Maine Number Theory Conference Theorem Refinement to arithmetic progressions: Let a; q be such that gcd(a; q) = 1. Then 1 π(x; q; a) := #fp ≤ x : p prime and p ≡ a mod qg ∼ Li(x): '(q) Background Results Sketch of Proof Motivation Theorem (Prime Number Theorem) π(x) := #fp ≤ x : p is primeg ∼ Li(x): Background Results Sketch of Proof Motivation Theorem (Prime Number Theorem) π(x) := #fp ≤ x : p is primeg ∼ Li(x): Theorem Refinement to arithmetic progressions: Let a; q be such that gcd(a; q) = 1. Then 1 π(x; q; a) := #fp ≤ x : p prime and p ≡ a mod qg ∼ Li(x): '(q) By restricting to the action of a congruence subgroup Γ ⊂ SL2(Z) of level N, we can associate that level to our modular form f (z). We say a modular form is a cusp form if it vanishes at the cusps of Γ; hence af (0) = 0 for a cusp form f (z). Background Results Sketch of Proof Modular Forms Recall that a modular form of weight k on SL2(Z) is a function f : H ! C with 1 X n 2πiz f (z) = af (n)q ; q = e n=0 and k f (γz) = (cz + d) f (z) for all γ 2 SL2(Z): We say a modular form is a cusp form if it vanishes at the cusps of Γ; hence af (0) = 0 for a cusp form f (z). Background Results Sketch of Proof Modular Forms Recall that a modular form of weight k on SL2(Z) is a function f : H ! C with 1 X n 2πiz f (z) = af (n)q ; q = e n=0 and k f (γz) = (cz + d) f (z) for all γ 2 SL2(Z): By restricting to the action of a congruence subgroup Γ ⊂ SL2(Z) of level N, we can associate that level to our modular form f (z). Background Results Sketch of Proof Modular Forms Recall that a modular form of weight k on SL2(Z) is a function f : H ! C with 1 X n 2πiz f (z) = af (n)q ; q = e n=0 and k f (γz) = (cz + d) f (z) for all γ 2 SL2(Z): By restricting to the action of a congruence subgroup Γ ⊂ SL2(Z) of level N, we can associate that level to our modular form f (z). We say a modular form is a cusp form if it vanishes at the cusps of Γ; hence af (0) = 0 for a cusp form f (z). A newform is a cusp form that is an eigenform for all Hecke operators. For a newform, the coefficients af (n) are multiplicative. We consider holomorphic cuspidal newforms of even weight k ≥ 2 and squarefree level N. Background Results Sketch of Proof Newforms We say f is a Hecke eigenform if it is a cusp form and Tnf = λ(n)f for n = 1; 2; 3;:::; where Tn is the Hecke operator. For a newform, the coefficients af (n) are multiplicative. We consider holomorphic cuspidal newforms of even weight k ≥ 2 and squarefree level N. Background Results Sketch of Proof Newforms We say f is a Hecke eigenform if it is a cusp form and Tnf = λ(n)f for n = 1; 2; 3;:::; where Tn is the Hecke operator. A newform is a cusp form that is an eigenform for all Hecke operators. We consider holomorphic cuspidal newforms of even weight k ≥ 2 and squarefree level N. Background Results Sketch of Proof Newforms We say f is a Hecke eigenform if it is a cusp form and Tnf = λ(n)f for n = 1; 2; 3;:::; where Tn is the Hecke operator. A newform is a cusp form that is an eigenform for all Hecke operators. For a newform, the coefficients af (n) are multiplicative. Background Results Sketch of Proof Newforms We say f is a Hecke eigenform if it is a cusp form and Tnf = λ(n)f for n = 1; 2; 3;:::; where Tn is the Hecke operator. A newform is a cusp form that is an eigenform for all Hecke operators. For a newform, the coefficients af (n) are multiplicative. We consider holomorphic cuspidal newforms of even weight k ≥ 2 and squarefree level N. The multiplicativity of the Ramanujan tau function follows from the fact that ∆(z) is a newform. Conjecture (Lehmer) For all n ≥ 1; τ(n) 6= 0. Background Results Sketch of Proof The Ramanujan Tau Function Ramanujan tau function: 1 1 Y X ∆(z) := q (1−qn)24 = τ(n)qn = q−24q2+252q3+··· : n=1 n=1 Conjecture (Lehmer) For all n ≥ 1; τ(n) 6= 0. Background Results Sketch of Proof The Ramanujan Tau Function Ramanujan tau function: 1 1 Y X ∆(z) := q (1−qn)24 = τ(n)qn = q−24q2+252q3+··· : n=1 n=1 The multiplicativity of the Ramanujan tau function follows from the fact that ∆(z) is a newform. Background Results Sketch of Proof The Ramanujan Tau Function Ramanujan tau function: 1 1 Y X ∆(z) := q (1−qn)24 = τ(n)qn = q−24q2+252q3+··· : n=1 n=1 The multiplicativity of the Ramanujan tau function follows from the fact that ∆(z) is a newform. Conjecture (Lehmer) For all n ≥ 1; τ(n) 6= 0. By the Deligne bound, (k−1)=2 af (p) = 2p cos(θp) for some angle θp 2 [0; π]. Natural question: What is the distribution of the sequence fθpg? Background Results Sketch of Proof The Sato-Tate Law Theorem (Deligne, 1974) If f is a newform as above, then for each prime p we have k−1 jaf (p)j ≤ 2p 2 . Natural question: What is the distribution of the sequence fθpg? Background Results Sketch of Proof The Sato-Tate Law Theorem (Deligne, 1974) If f is a newform as above, then for each prime p we have k−1 jaf (p)j ≤ 2p 2 . By the Deligne bound, (k−1)=2 af (p) = 2p cos(θp) for some angle θp 2 [0; π]. Background Results Sketch of Proof The Sato-Tate Law Theorem (Deligne, 1974) If f is a newform as above, then for each prime p we have k−1 jaf (p)j ≤ 2p 2 . By the Deligne bound, (k−1)=2 af (p) = 2p cos(θp) for some angle θp 2 [0; π]. Natural question: What is the distribution of the sequence fθpg? Background Results Sketch of Proof The Sato-Tate Law (Continued) Theorem(Barnet-Lamb, Geraghty, Harris, Taylor) new Let f (z) 2 Sk (Γ0(N)) be a non-CM newform. If F : [0; π] ! C is a continuous function, then 1 X Z π lim F (θp) = F (θ)dµST x!1 π(x) p≤x 0 2 2 where dµST = π sin (θ)dθ is the Sato-Tate measure. Further πf ;I (x) := #fp ≤ x : θp 2 I g ∼ µST (I )Li(x): From this normalization, we have Y −1 −1 L(s; f ) = 1 − eiθp p−s 1 − e−iθp p−s ; p and the n-th symmetric power L-function 0 n 1 0 1 −1 n Y Y ijθp (j−n)iθp −s Y −1 L (s; Sym f ) = @ 1 − e e p A @ Lp(s) A p-N j=0 pjN To pass to arithmetic progressions, we consider L(s; Symnf ⊗ χ). Background Results Sketch of Proof Symmetric Power L-functions We begin by writing 1 1 X m X k−1 m f (z) = af (m)q = m 2 λf (m)q : m=1 m=1 To pass to arithmetic progressions, we consider L(s; Symnf ⊗ χ). Background Results Sketch of Proof Symmetric Power L-functions We begin by writing 1 1 X m X k−1 m f (z) = af (m)q = m 2 λf (m)q : m=1 m=1 From this normalization, we have Y −1 −1 L(s; f ) = 1 − eiθp p−s 1 − e−iθp p−s ; p and the n-th symmetric power L-function 0 n 1 0 1 −1 n Y Y ijθp (j−n)iθp −s Y −1 L (s; Sym f ) = @ 1 − e e p A @ Lp(s) A p-N j=0 pjN Background Results Sketch of Proof Symmetric Power L-functions We begin by writing 1 1 X m X k−1 m f (z) = af (m)q = m 2 λf (m)q : m=1 m=1 From this normalization, we have Y −1 −1 L(s; f ) = 1 − eiθp p−s 1 − e−iθp p−s ; p and the n-th symmetric power L-function 0 n 1 0 1 −1 n Y Y ijθp (j−n)iθp −s Y −1 L (s; Sym f ) = @ 1 − e e p A @ Lp(s) A p-N j=0 pjN To pass to arithmetic progressions, we consider L(s; Symnf ⊗ χ). Rouse and Thorner (2017): under certain analytic hypotheses on the symmetric power L-functions, 3x3=4 log log x 202x3=4 log q(f ) jπ (x)−µ (I )Li(x)j ≤ 3:33x3=4− + f ;I ST log x log x for all x ≥ 2, where q(f ) = N(k − 1) Rouse-Thorner also leads to an explicit upper bound for the Lang-Trotter conjecture, which predicts the asymptotic of the number of primes for which af (p) = c for a fixed constant c.
Details
-
File Typepdf
-
Upload Time-
-
Content LanguagesEnglish
-
Upload UserAnonymous/Not logged-in
-
File Pages40 Page
-
File Size-