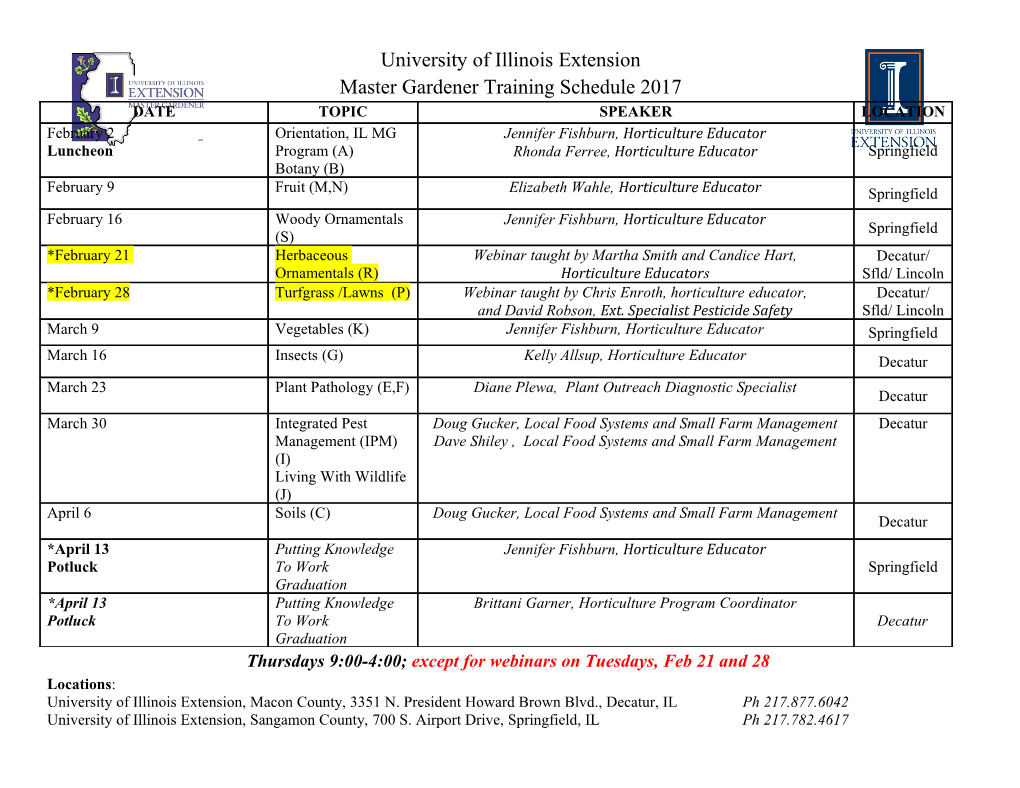
Draft Version 1.5: June 17, 2010 The GlueX Experiment in Hall-D The GlueX Collaboration∗ The goal of the GlueX experiment is to provide critical data needed to address one of the outstand- ing and fundamental challenges in physics { the quantitative understanding of the confinement of quarks and gluons in quantum chromodynamics (QCD). Confinement is a unique property of QCD and understanding confinement requires an understanding of the soft gluonic field responsible for binding quarks in hadrons. Hybrid mesons, and in particular exotic hybrid mesons, provide the ideal laboratory for testing QCD in the confinement regime since these mesons explicitly manifest the gluonic degrees of freedom. Photoproduction is expected to be particularly effective in producing exotic hybrids but there is little data on the photoproduction of light mesons. GlueX will use the coherent bremsstrahlung technique to produce a linearly polarized photon beam. A solenoid-based hermetic detector will be used to collect data on meson production and decays with statistics after the first full year of running that will exceed the current photoproduction data in hand by several orders of magnitude. These data will also be used to study the spectrum of conventional mesons, including the poorly understood excited vector mesons. In order to reach the ideal photon energy of 9 GeV for this mapping of the exotic spectrum, 12 GeV electrons are required. This document updates the physics goals, the beam and apparatus of the GlueX detector in Hall-D since the original proposal was presented to PAC 30 in 2006 [1]. I. INTRODUCTION Thus, their unambiguous identification is complicated by the fact that they can mix with qq¯. Excitations of the Quantum chromodynamics (QCD) provides a clear de- gluonic field binding the quarks can also give rise to so- scription of the strong interaction of quarks and gluons called hybrid mesons that can be viewed as bound states at high energy; however, obtaining quantitative predic- of a quark, antiquark and valence gluon (qqg¯ ). tions from QCD at low energy remains challenging. In- terestingly, it is at these low energies that we observe the most obvious physical manifestation of QCD, the spec- An alternative picture of hybrid mesons, one sup- trum of particles that make up the universe we live in, ported by lattice QCD [2], is one in which a gluonic baryons and mesons. While models do provide predic- flux tube forms between the quark and antiquark and tions for this spectrum, to obtain this spectrum as a so- the excitations of this flux tube lead to so-called hybrid lution to QCD is currently only possible using numerical mesons. Conventional qq¯ mesons arise when the flux techniques to calculate it, lattice QCD. tube is in its ground state, while hybrid mesons arise The observation, nearly five decades ago, that mesons when the flux tube is excited. In many models, some are grouped in nonets, each characterized by unique val- PC hybrid mesons can have a unique signature, exotic (not ues of J spin (J), parity (P ) and charge conjugation allowed for in a simple qq¯ system) J PC s. This signature (C) quantum numbers led to the development of the simplifies the spectroscopy of these exotic hybrid mesons quark model. Within this picture, mesons are bound because they do not mix with conventional qq¯ states. states of a quark (q) and antiquark (¯q). The three light- Lattice calculations presented in section III support the quark flavors (up, down and strange) suffice to explain existence of exotic-quantum-number states withing the the spectroscopy of most but not all of the charmless meson spectrum, independent of specific models. mesons lighter than thecc ¯ ground state (≈ 3 GeV/c2). Our understanding of how quarks form mesons has evolved within QCD and we now expect a richer spec- trum of mesons that takes into account not only the The GlueX detector (shown in Figure 1) has been de- quark degrees of freedom but also the gluonic degrees of signed to observe these exotic-quantum-number hybrid freedom. Gluonic mesons with no quarks (glueballs) are states . A program in spectroscopy, supported by a so- expected. Unfortunately, since the expected quantum phisticated amplitude analysis, will map out the spec- numbers of low-lying glueballs (below 4 GeV/c2) are not trum of the exotic-quantum-number states. At the same exotic, they should manifest themselves as extraneous time, the spectrum of the normalqq ¯ mesons will be stud- states that cannot be accommodated within qq¯ nonets. ied. Detailed comparisons of our experimental results to theoretical predictions on the excitations of the glu- onic field in mesonic systems will lead to a more detailed understanding of the role of glue in the confinement of ∗ Spokesperson:Curtis A. Meyer, ([email protected]) quarks inside hadronic matter. State Mass (GeV) Width (GeV) π1(1400) 1:351 ± 0:03 0:313 ± 0:040 π1(1600) 1:662 ± 0:015 0:234 ± 0:050 π1(2015) 2:01 ± 0:03 0:28 ± 0:05 State Production Decays − − z 0 z π1(1400) π p,¯pn π η ,π η − 0 z π1(1600) π p,¯pp η π,b1π,f1π,ρπ − π1(2015) π p b1π,f1π State Experiments π1(1400) E852, CBAR π1(1600) E852, VES, COMPASS, CBAR π1(2015) E852 TABLE I. The three exotic-quantum-number states for which some experimental evidence exists. The masses FIG. 1. A cut-away view showing the GlueX detector. and widths are the Particle Data Group average [8]. The See the text in section VI for a description of the com- decays with a superscript z are considered controversial. ponents. set of the known π2 decays in the analysis [7]. While II. MESON SPECTROSCOPY AND THE the VES experiment observed the π1(1600) in its other SEARCH FOR QCD EXOTICS three observed decay modes, they were never able to con- firm the 3π decay of the π1(1600) (even with extremely large statistics) [9]. Recently, the COMPASS experi- Despite an active experimental program, data sup- ment at CERN published their first results 3π final states porting the existence of these states are still sparse. Re- produced in peripheral pion production on nuclear tar- cent review articles [3{5] provide a summary of the field. gets [10, 11]. They report a robust signal for the π1(1600) This can be summarized as evidence exists for up to three in 3π, which is shown in Figure 2. Their analysis ap- PC −+ isospin-one, J = 1 , exotic-quantum-number states: pears to include all the decays of the π2(1670), but the π1(1400), π1(1600) and π1(2015) (whose properties are published information is still limited. Their results are summarized in Table I). Of these states, the π1(1400) also somewhat surprising in that both the π2(1670) and may not be a resonance, and if it is resonant, it is al- the π1(1600) have virtually the same mass and width. most certainly not a hybrid meson. Rather, it is more This is most clearly seen by the lack of phase motion be- naturally explained as a four-quark object [6]. tween these two states, shown in Figure 2, and leads to The π1(1600) and the π1(2015) are both potential can- concerns that the exotic state may be the result of un- didates for hybrid mesons. The π1(1600) suffers from a accounted for feed-through from the stronger π2 state, number of inconsistencies in its production, which ap- possibly caused by the use of the isobar model. pears different depending on how it decays. There is In photoproduction, the CLAS collaboration has car- also a good deal of controversy about its ρπ decay, with ried out the first search for the π1(1600) using a 4−5 GeV the existence of the decay mode apparently strongly de- photon beam and looking in the 3π final state. They see pendent on assumptions in the analysis. However, the no evidence for the π1(1600) in their analysis [12]. This number and variety of the experimental results suggest result could indicate that the π1(1600) does not decay to that this state does exists. The highest-mass state is the 3π, it is not produced in photoproduction, or both. In result of very-low statistics data sets from a single exper- summary, while the π1(1600) appears reasonably solid 0 iment (E852), and needs confirmation. Either of these via its other decay modes (η π, b1π and f1π), the simple higher-mass states are consistent with lattice predictions 3π mode has been called into question and needs clarifi- for the mass of the π1 hybrid. If both of these states are cation. confirmed, they could be explained as the ground and While there is evidence for the isovector member of −+ first-excited states of the 1 system. A result which the J PC = 1−+ nonet, we also expect two isoscalar states 0 may be consistent with recent lattice calculations dis- (η1 and η1), as well as two additional exotic nonets with cussed below. J PC = 0+− and 2+−. There is still no experimental As noted above, the ρπ (3π) decay mode of the evidence for any of these other states. The former are π1(1600) remains controversial. In 2005, a high-statistics necessary to establish the nonet nature of the π1 states, analysis of E852 data showed that the signal for the and the latter are expected in most models of hybrids. π1(1600) could be attributed to feed-through from the Table II lists the nine expected exotic-quantum number well-established π2(1670) due to the use of an incomplete states as well as model predictions for their widths and 2 Name JPC Total Width MeV Large Decays ) 2 -+ + PSS IKP 800 1 1 ρπ P COMPASS 2004 −+ - - - + π1 1 81 − 168 117 b1π, ρπ, f1π, a1η 700 π Pb → π π π Pb −+ 0.1 < t’ < 1.0 GeV 2/c2 η1 1 59 − 158 107 a1π, f1η, π(1300)π 600 0 −+ m l ∗ η1 1 95 − 216 172 K1 K, K1K, K K +− 500 b0 0 247 − 429 665 π(1300)π, h1π +− 400 h0 0 59 − 262 94 b1π, h1η, K(1460)K Intensity / (40 MeV/c 0 +− l 300 h0 0 259 − 490 426 K(1460)K, K1K, h1η +− b2 2 5 − 11 248 a2π, a1π, h1π 200 +− h2 2 4 − 12 166 b1π, ρπ 100 0 +− m l ∗ h2 2 5 − 18 79 K1 K, K1K, K2 K 0 0.6 0.8 1 1.2 1.4 1.6 1.8 2 2.2 2.4 TABLE II.
Details
-
File Typepdf
-
Upload Time-
-
Content LanguagesEnglish
-
Upload UserAnonymous/Not logged-in
-
File Pages10 Page
-
File Size-