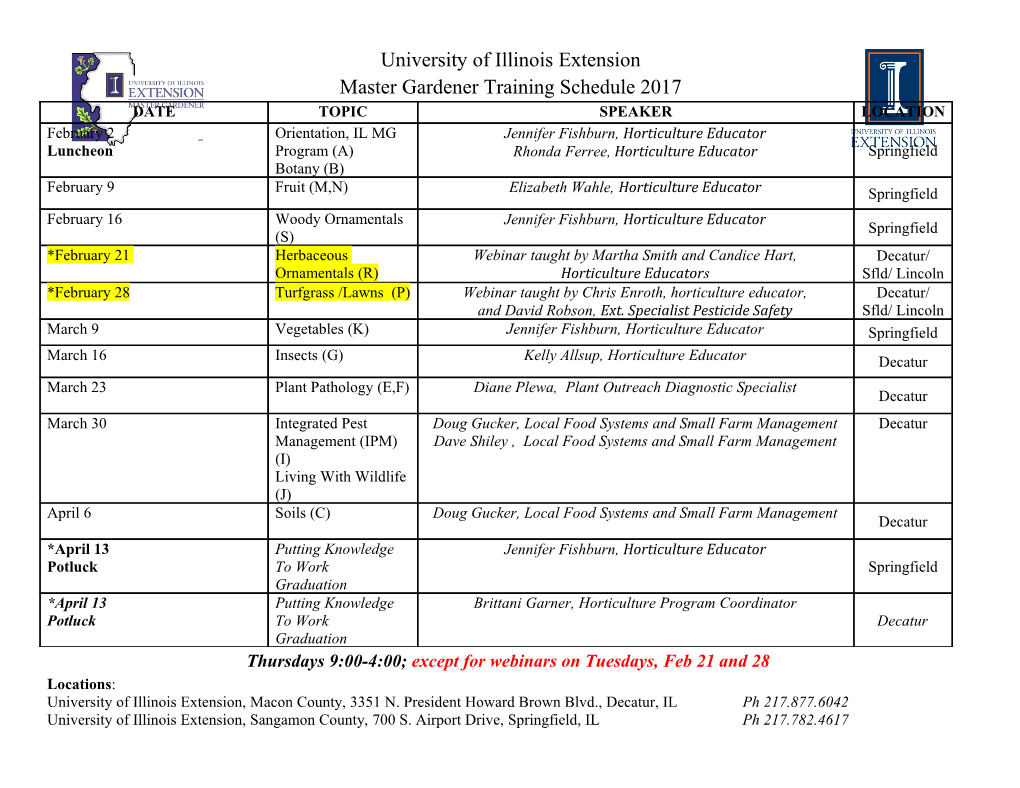
NOTE: To make this publication searchable, the text in this pdf was scanned from the original out-of-print publication. It is possible that the scanning process may have introduced text errors. If there is a question about accuracy, please check a copy of the orginal printed document at libraries or download an electronic, non- searchable version from the Pell Depository: http://nsgd.gso.uri.edu/aqua/ mdut81003.pdf Biology It’s a wery remarkable circumstance, sir,” said Sam, “that poverty and oysters always seems to go togeth - er.” “I don’t understand, Sam,” said Mr. Pickwick. “What I mean, sir,” said Sam, “is, that the poorer a place is, the greater call there seems to be for oysters. Look here, sir; here’s a oyster stall to every half- dozen houses. The streets lined vith ‘em. Blessed if I don’t think that ven a man’s wery poor, he rushes out of his lodgings and eats oysters in reg’lar despera - tion.” —Pickwick Papers, C. Dickens (1836) Biology of the Oyster Sam Weller was speaking of the flat oyster (Ostrea edulis) and the poor in England. At that time, about 500 million oysters were sold at Billingsgate every year, resulting in a cheap and readily available foodstuff (Gross and Smyth 1946). However, the production of the fishery declined throughout Europe until the oys- ter became too expensive for the poor. Eventually, flat oysters were maintained mainly by culture in areas representing only a portion of their former range. In discussing this decline, Gross and Smyth (1946) felt that two major and interre- lated causes for the decline were (1) overfishing and (2) the resultant conse- quence of a severely reduced population of oysters. Briefly, unrestrained fishing led to a severe reduction of oyster populations, the loss of young and immature oysters, and destruction of oyster beds. The decline was exacerbated by pollution. Cultivation of beds was initiated, but the resource remained depleted. Although a variety of factors were implicated in this lack of response to cultivation, Gross and Smyth (1946) felt that, because flat oyster populations had been depleted so greatly, they were thus much less resilient to various adverse environmental fac- tors. They postulated that the flat oyster populations had been so ravaged as to fall below a critical minimum, with the trend towards extinction continuing even after the fishing mortality was lessened. It is not clear if the eastern oyster would be similarly susceptible to over- fishing and environmental degradation. Though there is still much cause for con- cern, we believe that the Chesapeake Bay oyster resource might be more resilient. But that resilience, we also believe, depends on effective management strategies. We are convinced that the best management of any natural resource occurs when there is linkage between gathering of information by field or laboratory study and 2 Biology of the Oyster subsequent application of this information in resource management. Optimal management depends upon thorough understanding of the biology of the resource. We begin, then, with a general outline of oyster biology, emphasizing Crassostrea virginica and noting those areas requiring further research. Unless oth- erwise indicated, the word “oyster” refers to Crassostrea virginica. This section of the review considers the general biology and ecology of the eastern oyster, Crassostrea virginica, with comparative information for other species provided on occasion. Some aspects of oyster biology (e.g., genetics, effects of heavy metals) which have recently been reviewed elsewhere are dealt with briefly to avoid unnecessary repetition. Those matters which bear further investigation, either for academic interest or because of their practical (applied) importance, are so indicated. During the review, the reader should remember that reports of oyster performance under certain environmental conditions may reflect only the response of the local population being studied. It is possible that such responses might have been different if oysters from more northerly or southerly populations, or from different conditions of salinity, turbidity, etc., had been studied. Eastern oysters generally inhabit dynamic estuarine environments and are broadly eurytopic. Thus, that which holds for oysters taken from one environment and tested under controlled laboratory conditions may not hold for oysters from a different environment. Indeed, the topic of physiological adapta- tions of oysters throughout their broad distributional range has only been inves- tigated cursorily and might be a productive area for further research. ENVIRONMENTAL FACTORS Temperature Oysters are ectotherms (poikilotherms), ranging in distribution from the Gulf of St. Lawrence in Canada to the Gulf of Mexico and the West Indies (Abbott 1975). Thus they are probably subject to a temperature range of about 0°C or slightly below to about 36°C, although oysters exposed in air at low tide in southern regions have attained body temperatures of 46°-49°C (Galtsoff 1964). Copeland and Hoese (1966) reported apparent high-temperature-related mortality of intertidal oysters in central Texas. Crassostrea virginica has a maximum rate of ciliary activity of about 25°- 26°C. Above 32°C, ciliary activity is disrupted, whereas feeding may cease below 6°-7°C (Galtsoff 1964, but see Loosanoff 1958 below). Nelson (1928a) demon- strated that in New Jersey waters ciliary activity and shell opening virtually ceased below 5.6°C. Heartbeat activity is also temperature dependent (Fen” 1965), with frequency of beat highest at 24°C, declining steadily until reaching 10°C. Collier (1954) recorded shell movement and pumping rate of 66 oysters over long periods of time (from 3-24 weeks) and related his findings to tempera- ture. He concluded that C. virginica is fundamentally a cool-water animal with an optimum temperature range for pumping, growth, and survival of 15° to 25°C. Biology of the Oyster 3 Loosanoff (1958) studied oyster behavior at different temperatures. Some oysters from Long Island Sound pumped at temperatures as low as 1°C. Fifteen percent of those held between 2°-3°C and 50% of those held between 3°-4°C formed pseudofeces. However pumping rates were low below 8°C, increasing as temperatures rose to 16°C and maintaining a fairly constant rate between 16° and 28°C. There was a further rate increase as temperature rose to 32°C, with the rate dropping at temperatures above 34°C. A maximum pumping rate of 37,500 cc h-1 occurred at 24°C. Loosanoff noted that oysters were able to adjust rapidly to temperature change. Given the differences in temperature effects on ciliary activity, pumping and feeding noted by different authors, it is possible that oysters in different parts of their range have different environmental tolerances. Effects of elevated temperatures have been studied with regard to shell depo- sition, gonadal development, and biochemical constituents of oyster bodies (Ruddy et al. 1975). Oysters grown in the warm-water effluent of a power plant (temperatures ranged from 7.0 to 12.4°C higher than ambient) produced thicker shells and developed gonad four months earlier than did control oysters held near the power plant intake. In spite of the accelerated gonadal ripening, spawning occurred just one month earlier among the oysters in the heated effluent. In win- ter and spring, oysters in the effluent had higher concentrations of protein and carbohydrate and a higher condition index than did the control oysters. In sum- mer, there was no difference in condition index or protein and carbohydrate con- centrations between the experimental and control groups, although in some cases in late summer, control oysters were slightly superior to experimental oysters in these measures. This could indicate that the artificial elevation of temperature over summer extreme temperatures might be unfavorable (see also Quick 1971). With regard to reproduction, as temperature increases in spring, gametoge- nesis accelerates, resulting in development and maturation of sperm and eggs and thickening of gonadal epithelium (Kennedy and Battle 1964). Spawning of ripe gonads may be initiated as a result of a rapid rise in temperature (but see section on Reproduction). The spawning of oysters in hatcheries and laboratories both out-of-season and in-season depends on this reaction to temperature increase (Loosanoff and Davis 1963). In cool northern waters, the reproductive season is truncated; it may last only a few weeks. In warm southern waters, spawning may be spread out over most of the year. This subject is discussed more completely in the section on Reproduction. Temperatures lethal to oysters were determined by Henderson (1929) and Fingerman and Fairbanks (1957), but their experimental procedures did not pro- duce data that were ecologically useful. No other studies on temperatures lethal to adults, or to spat, have been reported for C. virginica. However, some research has been done on temperature and larval survival. Hidu et al. (1974) subjected 4 Biology of the Oyster fertilized eggs, ciliated gastrulae, and two-day-old veliger larvae to temperature increases for periods from 10 seconds to 16 hours. Mortality increased with increasing temperature and exposure time. Fertilized eggs were least resistant to higher temperatures, followed by ciliated gastrulae; veliger larvae were most tol- erant. Diaz (1973) used techniques different from Hidu et al. (1974) for exposing developmental stages for five seconds to 10°, 15° and 20°C increases. He report- ed that mortalities 48 h after exposure were higher for older stages, but he per- formed no tests for statistical significance. In nature, temperature influences larval development. For example, in Bideford River, Canada, Medcof (1939) found that the time it took oyster larvae to reach 365 µm in length depended on water temperature: 30 days at 19°, 26 days at 20°, and 24 days at 21°C. Davis and Calabrese (1964) found the temper- ature range for maximum growth for C. virginica larvae to be between 30.0 and 32.5°C at salinities ranging between 10.0 and 27.5 ppt.
Details
-
File Typepdf
-
Upload Time-
-
Content LanguagesEnglish
-
Upload UserAnonymous/Not logged-in
-
File Pages98 Page
-
File Size-