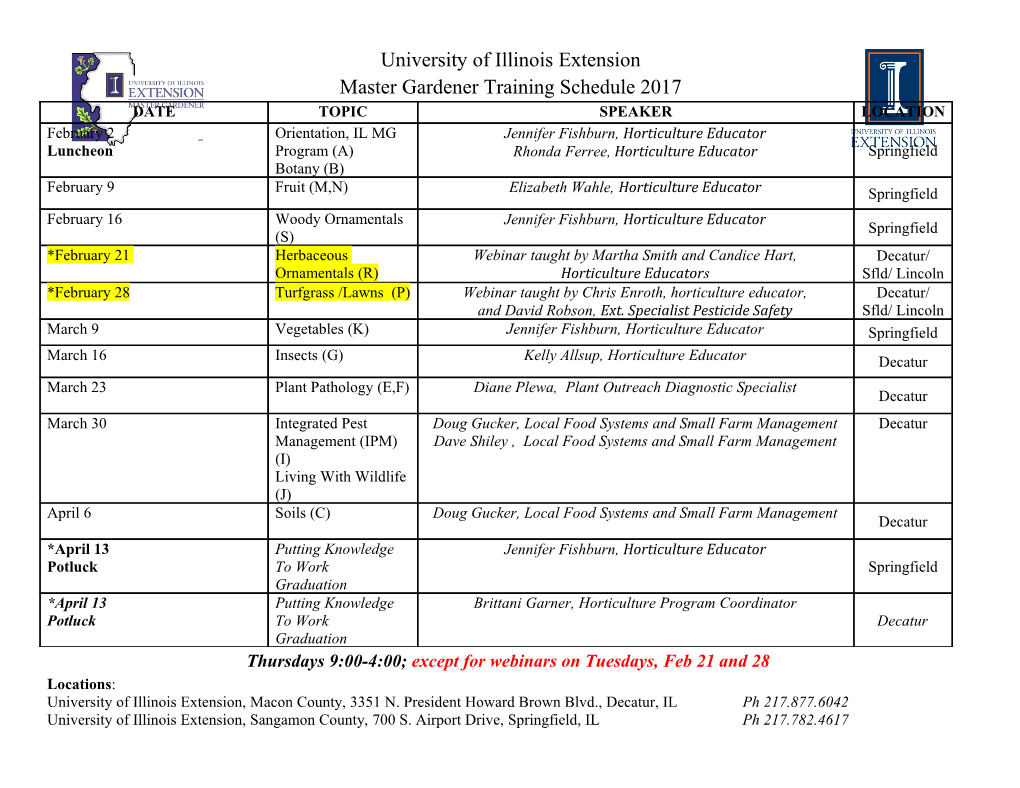
Observation of water dangling OH bonds around dissolved nonpolar groups P. N. Pereraa, K. R. Fegaa, C. Lawrenceb, E. J. Sundstroma, J. Tomlinson-Phillipsa, and Dor Ben-Amotza,1 aDepartment of Chemistry, Purdue University, West Lafayette, IN 47907; and bDepartment of Chemistry, Grand Valley State University, Allendale, MI 49401 Edited by Michael D. Fayer, Stanford University, Stanford, CA, and approved June 12, 2009 (received for review April 3, 2009) We report the experimental observation of water dangling OH spectra (20), and here, we employ it to uncover dangling OH bonds in the hydration shells around dissolved nonpolar (hydro- vibrational bands arising from the hydration shells around dissolved carbon) groups. The results are obtained by combining vibrational nonpolar groups. (Raman) spectroscopy and multivariate curve resolution (MCR), to The remainder of this article begins by describing the results reveal a high-frequency OH stretch peak arising from the hydration of the experiments we performed to confirm our assignment of shell around nonpolar (hydrocarbon) solute groups. The frequency the observed peak to water dangling OH bonds. Changes in the and width of the observed peak is similar to that of dangling OH intensity of the dangling OH peak are then used to estimate the bonds previously detected at macroscopic air–water and oil–water number of water dangling OH bonds around hydrocarbon chains interfaces. The area of the observed peak is used to quantify the of different size. The width of the observed dangling OH peak number of water dangling bonds around hydrocarbon chains of is used to establish a lower bound on the lifetime of the different length. Molecular dynamics simulation of the vibrational associated hydration-shell structure. Observed differences be- spectra of water molecules in the hydration shell around neopen- tween the average force along the dangling OH bond induced by tane and benzene reveals high-frequency OH features that closely saturated (alkane) and unsaturated (aromatic) hydrocarbon resemble the experimentally observed dangling OH vibrational groups are shown to be consistent with molecular dynamics bands around neopentyl alcohol and benzyl alcohol. The red-shift simulation predictions and previous experimental studies of -H ؊ of Ϸ50 cm 1 induced by aromatic solutes is similar to that previ- bonding in benzene–water clusters. Experimental and simula- ously observed upon formation of a -H bond (in low-temperature tion details are provided in Materials and Methods. benzene–water clusters). Results hydrophobic ͉ interface ͉ vibration ͉ Raman Fig. 1 shows representative examples of raw Raman spectra ob- tained from a series of aqueous neopentanol (CH3)3CCH2OH, hanges in the structure and dynamics of water induced by solutions ranging in concentration from 0 to 0.37 M. The broad Cnonpolar groups have long been considered to play a key role OH band peaked at Ϸ3,400 cmϪ1 in all these spectra appears in protein folding, ligand binding, and the formation of biological essentially identical to that of bulk water (Fig. 1, blue), except for cell membranes (1). Early thermodynamic evidence suggested the emergence of the solute’s intramolecular CH stretch bands that water may form an ‘‘iceberg’’ or clathrate-like structure (at Ϸ2,900 cmϪ1) with increasing solute concentration. The around nonpolar molecules (2). Although no such rigid struc- solute-correlated spectrum (Fig. 1, red), which is obtained from tures are currently thought to form (3), recent experimental (4, these input spectra by using MCR (as described in Materials and 5) and theoretical (6) results indicate that the rotational mobility Methods), clearly reveals several small OH stretch features in the of water molecules is reduced around nonpolar solutes (relative 3,100-cmϪ1 to 3,700-cmϪ1 frequency range. Our assignment of to bulk water). Moreover, fundamental theoretical arguments the narrower high-frequency peak to a water dangling OH bond (and simulation measurements) imply that the size of a hydro- arising from the hydration shell around hydrocarbon groups is phobic group may play a critical role in dictating water structure supported by the following observations. (7). This has led to the provocative suggestion that the structure The narrow high-frequency OH peak of interest (see Fig. 1) of water around hydrophobic groups of nanometer (or greater) has a frequency of Ϸ3,661 Ϯ 2cmϪ1 and a width of Ϸ33 cmϪ1 size may bear some resemblance to that at a macroscopic and thus closely resembles dangling OH stretch peaks previously air–water interface (8). Although cohesive interactions between observed at macroscopic air–water and oil–water interfaces (15, water and nonpolar groups tend to suppress the formation of an 18). More specifically, the dangling OH peaks at a macroscopic interfacial vapor layer (8–11), recent experimental (and molec- air–water and oil–water interfaces are centered at 3,705 Ϯ 5 ular dynamics) studies indicate that the nonpolar binding cavity cmϪ1 and 3,674 Ϯ 3cmϪ1, respectively, and have full widths of  Ϫ in bovine -lactoglobulin is completely dehydrated in liquid Ϸ40 cm 1 (18). Thus, dispersive (van der Waals) interactions water (12). Moreover, both experimental and simulation evi- between a water dangling OH bond and an overlying oil layer dence suggests that water at nonpolar interfaces experiences lead to a slight red-shift (relative to an air–water interface) that significantly larger fluctuations than either bulk water or water is comparable in magnitude to the red-shift of the OH band that at hydrophilic interfaces (13, 14). Here, we present experimental we have observed in aqueous neopentanol solutions. evidence that reveals a similarity between the structure of water When water is replaced by D2O, the frequency of the narrow around dissolved hydrocarbon groups and that at macroscopic solute-correlated band is decreased by the expected factor of oil–water interfaces (15–18), in the sense that both interfaces approximately ͌2, whereas replacing all of the solute CH groups induce the formation of dangling OH bonds. by CD (and by using H O as the solvent) leaves the frequency of We have detected water dangling OH bonds by combining 2 vibrational Raman spectroscopy with multivariate curve resolution (MCR). This procedure is used to decompose solution spectra into Author contributions: D.B.-A. designed research; P.N.P., K.R.F., C.L., E.J.S., and J.T.-P. bulk water and solute-correlated spectral components—the latter performed research; P.N.P., K.R.F., E.J.S., and J.T.-P. analyzed data; and D.B.-A. wrote the of which includes hydration-shell vibrational features. Although, paper. MCR is a well-established method for quantifying mixture com- The authors declare no conflict of interest. positions (by using spectroscopic and/or chromatographic data) This article is a PNAS Direct Submission. (19), we have recently used this method to measure solvation-shell 1To whom correspondence should be addressed. E-mail: [email protected]. 12230–12234 ͉ PNAS ͉ July 28, 2009 ͉ vol. 106 ͉ no. 30 www.pnas.org͞cgi͞doi͞10.1073͞pnas.0903675106 Downloaded by guest on October 1, 2021 hydration-shell water molecules, rather than from the alcohol Aqueous OH head group. Neopentanol Solute- Raman Spectra Correlated The areas of the dangling OH peaks are found to scale [C] M Spectrum approximately linearly with solute concentration, confirming 0.00 that the dangling OH bonds are associated with isolated alkane 0.06 0.17 chains rather than aggregates consisting of 2 or more solutes. 0.26 Our observation of dangling OH bonds around solutes as small 0.37 Dangling OH Band as methanol (see dangling OH spectra in Fig. 2 Inset) clearly implies that liquid water does not form perfect clathrate–hydrate Intensity (relative) structures around small nonpolar groups. The integrated area of the dangling OH peak obtained from aqueous n-butanol is very similar to that observed around diethyl ether and tetrahydrofu- ran (of the same concentration). This implies that dangling OH 3000 3500 4000 bond formation is relatively insensitive to the secondary (con- -1 Vibrational Frequency (cm ) formational) structure of short alkane chains, because the latter 3 solutes all have 4 methyl (or methylene) groups. On the other Fig. 1. The broad OH stretch band of pure water (blue), obtained by using hands, the number of dangling OH bonds around neopentanol normal (nonenhanced) Raman scattering, is shown along with the corre- is approximately half that around N-pentanol (as shown in Fig. sponding spectra of aqueous neopentanol solutions of various concentrations 2), which implies that branching of longer-chain alkanes tends to [C]. The input spectra are normalized to unit area and analyzed by using MCR decrease the dangling OH formation probability. This conclu- to produce the (red) solute-correlated Raman spectrum, which reveals a sion is further supported by our observation that cyclohexanol narrow dangling OH peak at 3,661 Ϯ 2cmϪ1. induces approximately the same number of water dangling OH bonds as n-pentanol.* Moreover, our results pertaining to a the solute-correlated band unchanged. These results confirm series of n-alcohols (solid points in Fig. 2) suggest that there is that the observed solute-correlated band results from an OH a sharp increase in the dangling OH formation probability (OH stretch vibration (rather than a CH-related combination band). %) between n-butanol and n-pentanol (whose potential signif- Our assignment of this OH band to water, rather than the icance is further discussed below). CHEMISTRY alcohol OH head group, is supported by our observation of Previous studies have suggested that the OH stretch frequency shifts of water molecules in pure liquid water and aqueous similar solute-correlated OH bands in aqueous solutions of solutions are primarily dictated by the electric field (Stark shift) linear and cyclic ethers, diethyl ether CH CH OCH CH and 3 2 2 3 experienced by each OH group (21–23).
Details
-
File Typepdf
-
Upload Time-
-
Content LanguagesEnglish
-
Upload UserAnonymous/Not logged-in
-
File Pages5 Page
-
File Size-