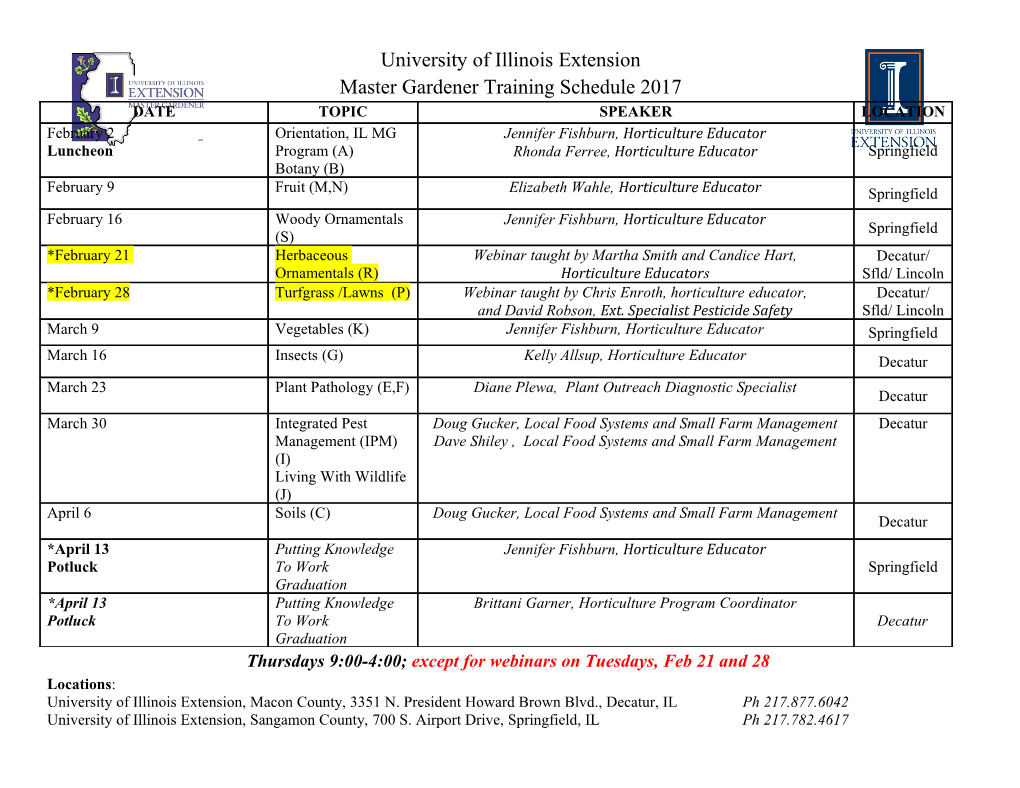
The ISME Journal (2015) 9, 2117–2127 & 2015 International Society for Microbial Ecology All rights reserved 1751-7362/15 www.nature.com/ismej ORIGINAL ARTICLE Feeding currents facilitate a mixotrophic way of life Lasse T Nielsen and Thomas Kiørboe Centre for Ocean Life, National Institute of Aquatic Resources, Technical University of Denmark, Charlottenlund, Denmark Mixotrophy is common, if not dominant, among eukaryotic flagellates, and these organisms have to both acquire inorganic nutrients and capture particulate food. Diffusion limitation favors small cell size for nutrient acquisition, whereas large cell size facilitates prey interception because of viscosity, and hence intermediately sized mixotrophic dinoflagellates are simultaneously constrained by diffusion and viscosity. Advection may help relax both constraints. We use high-speed video microscopy to describe prey interception and capture, and micro particle image velocimetry (micro-PIV) to quantify the flow fields produced by free-swimming dinoflagellates. We provide the first complete flow fields of free-swimming interception feeders, and demonstrate the use of feeding currents. These are directed toward the prey capture area, the position varying between the seven dinoflagellate species studied, and we argue that this efficiently allows the grazer to approach small-sized prey despite viscosity. Measured flow fields predict the magnitude of observed clearance rates. The fluid deformation created by swimming dinoflagellates may be detected by evasive prey, but the magnitude of flow deformation in the feeding current varies widely between species and depends on the position of the transverse flagellum. We also use the near-cell flow fields to calculate nutrient transport to swimming cells and find that feeding currents may enhance nutrient uptake by E75% compared with that by diffusion alone. We argue that all phagotrophic microorganisms must have developed adaptations to counter viscosity in order to allow prey interception, and conclude that the flow fields created by the beating flagella in dinoflagellates are key to the success of these mixotrophic organisms. The ISME Journal (2015) 9, 2117–2127; doi:10.1038/ismej.2015.27; published online 17 February 2015 Introduction 1998) that are thus constrained both by viscosity and diffusion. Many or most eukaryotic flagellates are in principle Dinoflagellates are one of the most abundant and mixotrophic, that is, they photosynthesize and take ecologically important groups of aquatic flagellates. up food simultaneously (Flynn et al., 2013). One Most of them are either heterotrophic or mixo- implication of this is that they need to be able to trophic (Stoecker, 1999). They are generally both harvest dissolved inorganic nutrients and 10–100 mm long, swim at 2–20 body lengths s À 1,and intercept and capture particulate prey. Nutrient hence operate at Reynolds numbers of 10 À 4–10 À 1. uptake in unicellular organisms is typically con- Thus, dinoflagellates are constrained by both diffu- strained by the diffusive delivery of nutrients to the sion and viscosity but have nevertheless evolved cell surface, and is much more efficient for small into a diverse and hugely successful group. What than for large cells (Munk and Riley, 1952; Fiksen has ensured this success? et al., 2013). Conversely, prey interception is Swimming will increase nutrient uptake by constrained by viscosity for small cells operating at renewing nutrient-replete water around the cell, low Reynolds numbers because the viscous boun- and swimming or feeding currents are often also dary layer surrounding the predator may push required to encounter prey. The enhancement of away the prey as it is approached (Kiørboe, 2011). nutrient delivery and the rate of prey encounter and Purely autotrophic organisms are, therefore, typically prey capture success may, however, depend on the very small (for example, cyanobacteria), and purely flow field that the flagellate generates (Langlois heterotrophic organisms typically larger, with the et al., 2009). The advective enhancement of nutri- mixotrophic strategy occurring mainly in interme- E ents because of swimming/feeding currents is diately sized ( 5–100 mm) organisms (Stoecker, quantified by the Sherwood number, with Sh ¼ 1 for no enhancement relative to pure diffusion (Karp- Boss et al., 1996). For an organism moved by a body Correspondence: LT Nielsen, Centre for Ocean Life, National force (such as gravity), Sherwood numbers are close Institute of Aquatic Resources, Technical University of Denmark, to unity for the Reynolds numbers considered here, Kavalerga˚rden 5, Charlottenlund 2920, Denmark. E-mail: [email protected] whereas it is higher for a self-propelled organism, as Received 29 December 2014; accepted 8 January 2015; published suggested by theoretical models based on idealized online 17 February 2015 flows (Magar et al., 2003; Magar and Pedley, 2005; Flow fields around swimming microorganisms LT Nielsen and T Kiørboe 2118 Langlois et al., 2009; Bearon and Magar, 2010). et al., 2014; Goldstein, 2015). We use micro particle Similarly, prey encounter by direct interception image velocimetry (micro-PIV) to visualize the flow depends on the flow field generated by the flagellate and high-speed video microscopy to describe prey and is higher the closer the streamlines come to the encounter and prey capture. We demonstrate that capture region on the flagellate (Langlois et al., feeding flows are sufficient to account for observed 2009). Interception feeding has been suggested as a clearance rates and that the flows generated differ common prey encounter mechanism in flagellates between species and likely represent adaptations to (Fenchel, 1982, 1984; Christensen-Dalsgaard and feeding on evasive and nonevasive prey. Finally, the Fenchel, 2003), but feeding flows of free-swimming flows imply significantly elevated nutrient uptake forms have not been described in any detail. Finally, rates relative to that because of pure diffusion. many of the potential prey of dinoflagellates are evasive, that is, they can perceive the flow dis- Materials and methods turbance generated by the approaching flagellate, and escape (see, for example, Jakobsen, 2001). Cultures and laboratory conditions Specifically, plankton organisms may respond to We studied seven species of mixotrophic and fluid deformation when it exceeds a certain thresh- heterotrophic dinoflagellates (Figure 1 and Table 1). old that typically is in the range of 1–10 s À 1 (Kiørboe Two of these species are peduncle feeders (Amphi- and Visser, 1999; Kiørboe et al., 1999; Jakobsen, dinium longum and Dinophysis acuta), whereas the 2001, 2002; Fenchel and Hansen, 2006). Thus, rest engulf particles directly. All experimental overall, there are tradeoffs between rapid swimming organisms were taken from our culture collection. to enhance nutrient uptake and prey encounter The cultures were grown in B1-medium with a versus the resulting elevated risk of eliciting prey salinity of 32 at 20 1C. Most of the mixotrophic escape responses, and these tradeoffs may depend species grew photoautotrophically at E100 mmol strongly on the flow and deformation fields gener- photons m À 2 s À 1. D. acuta is an obligate mixotroph ated by the dinoflagellate. and was fed the mixotrophic ciliate Mesodinium Most dinoflagellates have two distinct flagella, rubrum that, in turn, was fed the cryptophyte one trailing after the cell as it swims, termed the Teleaulax amphioxeia (Park et al., 2006). The longitudinal flagellum, and one encircling the cell, heterotrophic dinoflagellates were fed weekly with termed the transverse flagellum. The transverse Rhodomonas salina and presented with only low flagellum and the distal end of the longitudinal light level (E5 mmol photons m À 2 s À 1). flagellum are typically situated in grooves on the outside of the cell (termed the cingulum and sulcus, respectively). Combined, the two flagella propel the Experimental setup cell forward in a helical path (Fenchel, 2001). The Observations of swimming and feeding dino- position of the transverse flagellum varies between flagellates were made using an Olympus IX71 species; it is typically located equatorially, but inverted microscope (Olympus, Ballerup, Denmark) several species have it positioned more anteriorly equipped with 4–100  DIC objectives, two build-in (Figure 1). The different flagellar arrangements magnifying lenses (1–1.6  and 1–2  ) and a 2 3 À 1 likely create different flows in the near field region Phantom V210 high-speed (10 À 10 frames s ), of the cell, with possible implications for nutrient high-resolution (1280  800 pixels) video camera transport and perception and capture of prey. All capable of post-triggering (Vision Research, Wayne, dinoflagellates have but a single point of prey NJ, USA). Fields of view ranged from 0.64 mm  0.40 ingestion; direct engulfment takes place only mm when applying the 40  objective to through a single non-permanent cytostome, typi- 0.26 mm  0.16 mm when applying the 100  objec- cally located in the sulcus, and peduncles also tive. The organisms swam in 10 mm  10 mm cham- occupy a fixed position, also typically originating bers 0.5 mm high. These were produced using from the sulcus (see Figure 1) (Hansen and Calado, several layers of cover slip glass mounted on a 1999). It is unknown how dinoflagellates ensure that microscope slide with silicone grease. The micro- prey arrives to this particular region. It is also scope was focused in the middle of this
Details
-
File Typepdf
-
Upload Time-
-
Content LanguagesEnglish
-
Upload UserAnonymous/Not logged-in
-
File Pages11 Page
-
File Size-