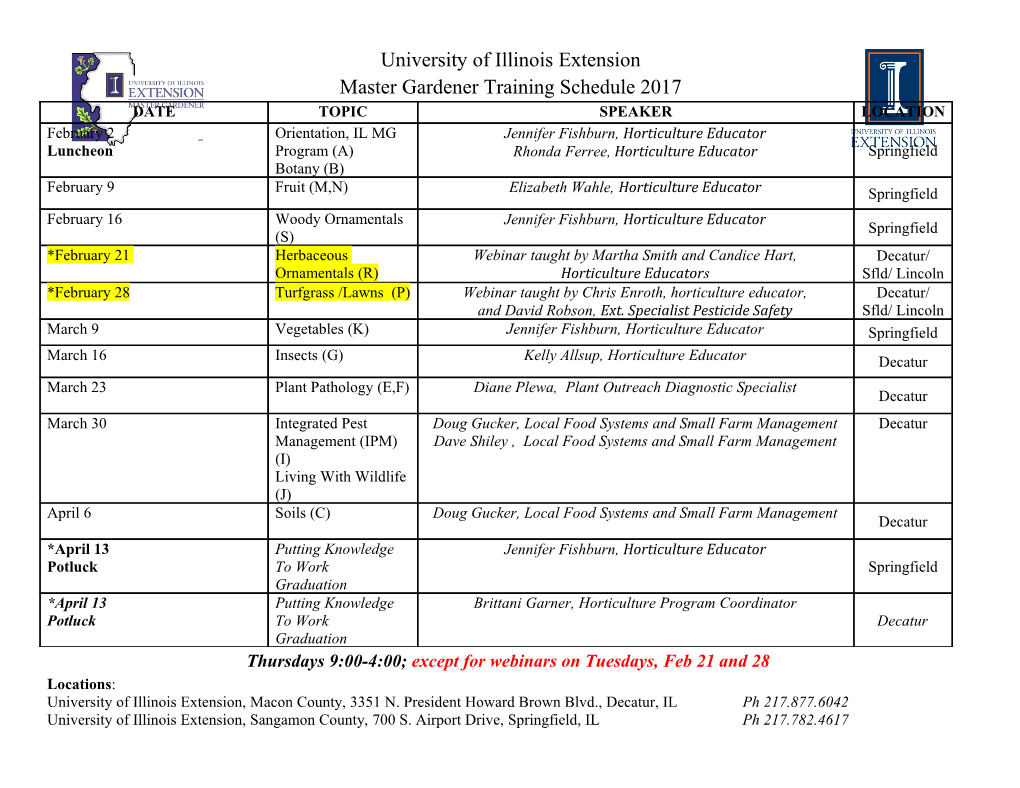
The Whipple Spacecraft - Amissiontostudythetrans-Neptunianpopulation Hannah M Calcutt Harvard-Smithsonian Center for Astrophysics 60 Garden Street, Cambridge, MA 02138, USA and School of Physics and Astronomy, University of Southampton, Southampton, SO17 1BJ, UK e-mail:[email protected] May 13, 2010 Abstract In response to the NASA discovery mission announcement of opportunity, we are developing aproposalfortheWhipplespacecraft.WhippleaimstosurveytheKuiperbelt,Sednaregion, and Oort cloud to look for small distant objects using the occultation technique. Here we present details of the mission and the results of a simulationofthespacecraftdetectorto quantitatively determine the limits of observation and therefore the scientific limits of the mission. It has been determined that Whipple can detect objects out to 20,000AU. In the Kuiper belt it will be able to detect objects as small as 300m indiameter,significantly advancing the current limits of observation in the region. IntheSednaregionitwilldetect objects as small as 1km in diameter which will allow other Sedna-like objects to be detected. In the Oort cloud it will be able to detect objects as small as 5km in diameter providing the first detections of Oort cloud objects. Contents 1Introduction 2 1.1 Background on the trans-Neptunian population . ........ 2 1.1.1 TheKuiperBelt ............................. 2 1.1.2 The Sedna Region . 4 1.1.3 The Oort Cloud . 4 1.2 The Occultation Technique . ... 6 1.3 Whipple’s Scientific Objectives . ...... 8 1.4 Instrumentation Requirements . ..... 9 1.5 OnBoardeventdetection ........................... .11 2SimulatinganOccultationevent 12 3ResultsfromtheWhippleCameraModel 18 3.1 TheKuiperBelt.................................. 18 3.1.1 The effectsofsamplingrate ....................... 20 3.1.2 The effects of detector read noise . .. 22 3.1.3 Implications for Whipple’s Science objectives . ......... 22 3.2 TheSednaRegion................................. 24 3.2.1 The effectsofsamplingrate ....................... 26 3.2.2 The effect of detector read noise . .. 28 3.2.3 Implications for Whipple’s science objectives . ......... 28 3.3 TheinnerOortCloud .............................. 30 3.3.1 The effectsofsamplingrate ....................... 32 3.3.2 The effects of detector read noise . .. 34 3.3.3 Implications for Whipple’s Science Objectives . ........ 34 4ConclusionsandFurtherWork 36 5Acknowledgements 37 1 Chapter 1 Introduction The Whipple spacecraft mission is a proposed discovery classmissiontosurveythetrans- Neptunian population of the Solar System. One of NASA’s key scientific goals is to advance the scientific knowledge of the origin and history of the SolarSystembylearninghowthe Sun’s family of planets and minor bodies originated (NASA strategic plan 2006)[28]. Whipple will be key in achieving this goal by dramatically improving our ability to observe the trans- Neptunian popultation. It will look for occultations of bright stars by small, distant objects to detect and measure the size distribution and heliocentricradialdistributionofobjects in the Kuiper belt, Sedna region and Oort cloud. This technique has been used previously to study the Kuiper belt and infer the collisional history of the region(Schlichting et al. (2009)[33] and Bianco et al. (2009)[5]). Previous surveys have however lacked the fast photometry necessary to observe very small (∼300m) objects whose occultation events have averyshortduration(∼1secondorless)andhavebeenhinderedbysmallfieldsofview.In the case of ground based surveys there is also the problem of high rates of false-positives due to atmospheric scintillation and terrestrial effects (bats,birds,andplanes).Whipplewill comprise of a small telescope in an Earth leading solar orbit with a wide field of view that will be able to image up to 40,000 stars simultaneously. This will allow Whipple to detect many occultations and expand our observational horizon to asfaroutastheOortcloud. Figure 1.1 shows the region of the Solar System that Whipple aims to study. 1.1 Background on the trans-Neptunian population 1.1.1 The Kuiper Belt As early as 1930 the possibility of other planets revolving around the Sun beyond Pluto was suggested (Leonard (1930) [26]), however it wasn’t untilthe1950sthatEdgeworthand Kuiper conjectured the existence of a belt of small bodies beyond Neptune. The first Kuiper belt object (KBO) was discovered in 1992 (Jewitt & Luu (1992) [22]) finally providing in- formation on orbital distances and absolute magnitudes for this class of object. Similar to the Asteroid belt, the Kuiper belt consists mainly of small bodies that are remnants of the formation of the Solar System composed of frozen volatiles (methane, ammonia, and water). The region spans from roughly 30AU to 55AU and is comprised of objects ranging in diam- eter from 100km to under 20km (Bernstein et al. (2004) [4]). 2 Figure 1.1: The region highlighted in red will be the focus of the Whipple mission. Source: TAOS, results from the first two years, Zhang et al. (2008)[36] Dynamical Structure The Kuiper belt can be separated into 4 sub classes; resonant objects, classical objects, the scattered disk and the extended scattered disk owing their respective dynamics. Resonant objects are objects that are trapped in mean-motion resonance with Neptune (The Solar System beyond Neptune (2008)[3]). Classical objects are those whose orbits have largely been undisturbed by Neptune. They can be further separated into hot and cold populations. The cold population are objects with low orbital eccentricities and inclinations as a result of the accretion disks at the time of formation of the Solar System. The hot population are objects with higher inclinations (up to 30 degrees) which arebelievedtohaveformednear Jupiter and dynamically ejected to their present orbits by the gas giants. Scattered disk objects have perihelia in the ∼35 to 40AU range and typically have large eccentricities and inclinations which is likely due to excitation by Neptune. The existence of a fourth class of object, the extended scattered disk, has only recently been conjectured due to the discov- ery of 2000 CR105.TheunusualorbitofCR105 places it dynamically beyond the scattered disk. Objects in this region would have large semi-major axes, eccentricities, and perihelion distances (Gladman et al. (2002)[19]). This complex dynamical structure indicates that the Kuiper belt has a complicated history of excitation which is difficult to explain if the outer planets have always had their present orbits. 3 Size Distribution The size distribution provides information on the formationoftheKuiperbeltandofthethe collisional history of the region. In the early Solar System planetesimals in the Kuiper belt collided and merged to form larger objects. As these objects grew their gravity increased the collisions between smaller objects until these collisions became destructive, causing a break in the size distribution of objects. The size distribution of large objects (∼100km and above) has been well determined through observation (Trujillo et al. (2001)[35], Gladman et al. (1998)[20], Chiang & Brown (1999)[11]) but determining the size distribution of smaller objects has been hindered by the faintness of objectsatKuiperbeltdistances.The 1−q −2.5 size distribution of large objects follows a power law of N>r ∝ r with q =5(N>r ∝ r ) where Nr is the number of KBOs larger than a size r (Bernstein et al. (2004)[4]). Simulations however show that for diameters <100km the size distribution becomes shallower and q = −2.5 3.5(N>r ∝ r )ascollisionsbecomedestructive(Davis&Farinella(1996)[15], Davis & Farinella (1997)[12], Kenyon et al. (2002)[24]). Pan & Sari (2004)[30], through simulation, found this break diameter to occur at 40km, smaller than the previous value of 70km found by Bernstein et al (2004)[4], giving a power law slope of # 3. Kenyon &Bromley (2004)[25] suggest that estimates of the break diameter depend stronglyontheinitialmassofthe Kuiper belt and on the amount of stirring by Neptune. They find abreakdiameterof ∼100m if there is a small initial mass in the Solar Nebula and no long-range stirring by Neptune but up to ∼30km if there is a large mass in the nebula and vigorous long-range stirring by Neptune. They also predict that their should be very few objects <1km due to collisional grinding. 1.1.2 The Sedna Region The Sedna region is defined by the position of the object 90377 Sedna which has a perihelion of 76AU and a semi-major axis of 495 AU (Trans Neptunian objects 2005[23]). It has such alargeperihelionthatislikelyduetoexcitationbyanearbypassingstarandnotby gravitational interactions with the giant planets (Brown etal.(2004)[8]).Thisunusualorbit makes it difficult to class Sedna as a member of the Kuiper belt however it may be the first observed object of the inner Oort cloud. 1.1.3 The Oort Cloud The Oort cloud was first proposed by Oort in 1950 to explain the energy distribution of the orbits of long-period comets (LPCs). One third of LPCs were found to be gravitational bound to the Sun but have semi-major axes >104AU as represented by the peak in figure 1.2. To explain this peak Oort suggested that the Sun was surrounded by a reservoir of comets containing about 1011 comets of observable size, spanning a region from about 50000AU out to 150000 AU (Oort 1950[29]). These comets are so far from the Sun that they are easily perturbed by passing stars and the tidal pull of the galactic disk (Byl (1983)[9], Heisler et al. (1986)[21]), as well as passing molecular clouds (Biermann (1978)[6], Bailey (1983)[2]).
Details
-
File Typepdf
-
Upload Time-
-
Content LanguagesEnglish
-
Upload UserAnonymous/Not logged-in
-
File Pages87 Page
-
File Size-