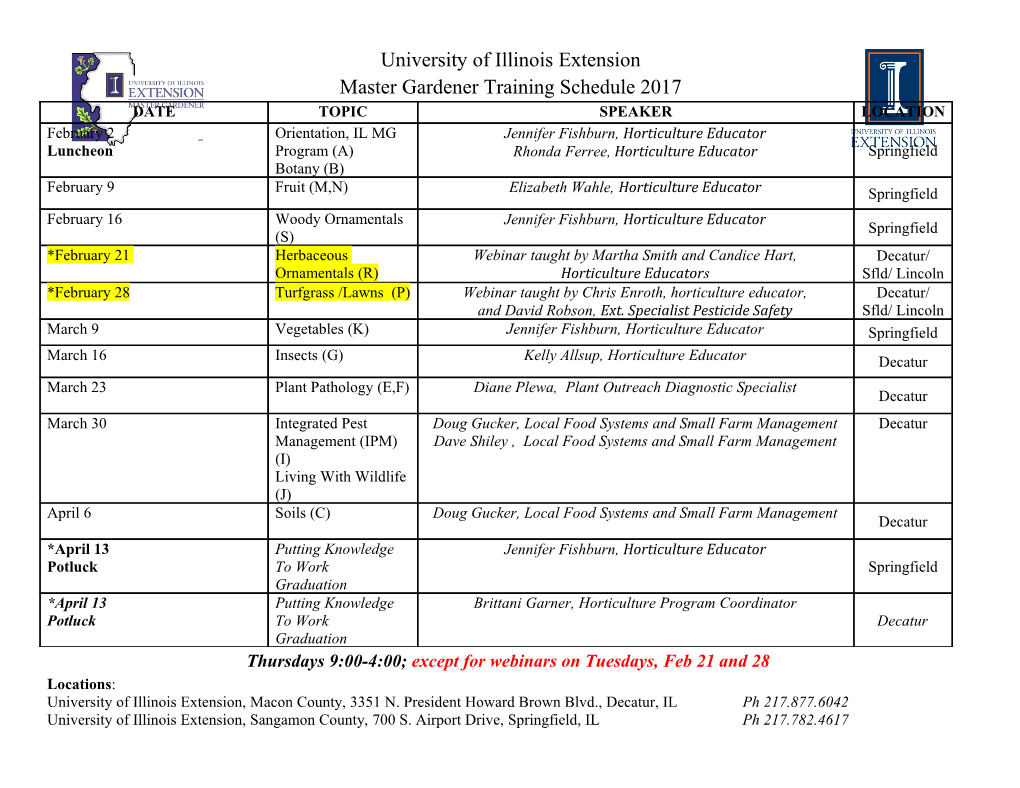
... ••........° ............ .•.. .. .. o . .°°°. •.°..- % .. .. .. o° .. .. .•. ••.° 1 i! NATIONAL AE RON, -J C-, AND SPACE ADMINISTRATIO N ..... •....... ......................S P L O 1 N E TG T O F M .... •....... ... ..... .......... • •....... .... :..'•..........-. .......-. o.. •... ...... ........... ........ o... ...........:::::t g •° .'.. o%%...... .. °........ :..:..........FR .... °....°o°. AIN R~I ...... .... Sp ngel V 2 1 FINAL REPORT PANEL 7 REACTION PROCESSES IN HIGH-PRESSURE FLUID SYSTEMS MAY 28, 1970 W. R. Downs Chairman, Panel 7 CONTENTS Section Page INTRODUCTION . .. ...... I SECTION A - DESCRIPTION OF TANKS IN USE ON THE APOLLO SPACECRAFT ............... ......... 3 SECTION B - METALLURGY - APOLLO HIGH PRESSURE VESSELS . 19 SECTION C - CHEMISTRY SURVEY ................ 33 SECTION D - THERMODYNAMICS RESTRICTIONS ON ENERGY PROCESSES IN THE APOLLO 13 CRYOGENIC OXYGEN TANK NUMBER 2 .......... ........ .. 83 SECTION E - SUMMARY . .. ....... .......... .I..101 SECTION F - FINDINGS .......... .......... ... 105 SECTION G - ACKNOWLEDGMENTS ...... ............. ... 107 APPENDIX A - COMMAND AND SERVICE MODULE FLUIDS ON SPACECRAFT 109 .......... ............. 109 APPENDIX B - CONTAMINANTS DETECTED AND IDENTIFIED IN GROUND SUPPORT EQUIPMENT ........ ............ 113 APPENDIX C - HARDWARE ANALYSIS OF P/N 15241-637 . ...... 123 APPENDIX D - THE POSSIBILITY OF A CATASTROPHIC PRESSURE FLUCTUATION IN A NEAR-CRITICAL-POINT FLUID ......... .............. ... 129 APPENDIX E - CHEMISTRY AND THERMOCHEMISTRY OF FLUIDS IN THE CRITICAL AND SUPERCRITICAL REGIONS . ..... 163 APPENDIX F - AN ASSESSMENT OF THE HAZARDOUS ASPECTS OF STORAGE AND HANDLING OF OXIDIZERS AND FUELS USED IN APOLLO AND IM SYSTEMS ........ 181 APPENDIX G - COMMENTS ON SUPERCRITICAL OXYGEN TANK WIRING CONDUIT .......... ...... ... 199 iii '£RWIDNG PAGp BLANK NOT FIln PANEL 7 - REACTION PROCESSES IN HIGH-PRESSURE FLUID SYSTEMS INTRODUCTION This report presents the results of the physical, metallurgical, chemical, and thermodynamic surveys that have been made on the high­ pressure tank and plumbing systems of the Apollo spacecraft. These systems comprise the pressurized oxygen, hydrogen, nitrogen, propellant, and helium tanks and related plumbing of the command, service, and lunar modules. The purposes of the surveys are to lend support to the Apollo 13 investigation, to provide review and to extend knowledge of spacecraft pressure systems, and to contribute to the safety of future manned space flights. To achieve these objectives, descriptive data for all tanks were collected, a metallurgical survey and a comprehensive physical chemical survey of all systems were made, and some detailed thermodynamical calculations were performed. The overall descriptive survey of the high-pressure tanks used on the Apollo spacecraft systems, including a review of the metallurgy involved in their construction, pointed to (1) the importance of a crit­ ical examination of any incompatibilities in materials selections and (2) the need for physical chemistry and catalysis studies of the reac­ tion processes involved in high-pressure fluid systems. The result is a critical assessment 'ofthe physical and chemical processes that can occur in high-pressure fluids and an assessment of the hazards and pos­ sible failure mechanisms associated with containment of cryogenic mate­ rials. Findings based on these surveys are tabulated in the report. As a general point, it should be noted that there is no such thing as a nonreactive substance. The stability of a substance in its envi­ ronment is a matter of degree. Theoretically, the reactivity of mate­ rials can be assessed in terms of entropy and energy changes along with an implied characteristic time. In practice, either in engineering or in science, the experiment and the insight into the basic phenomena provide the real assessment of reactivity. The successful completion of two lunar landings and, before those, the extensive design, develop­ ment, and qualification aspects of the Apollo Program can be thought of as experimental verifications. The Apollo 13 incident provides an opportunity for reexamination, objective and refined evaluation, im­ proved design, and establishment of a broader base for future technolog­ ical objectives. An independent review of reaction processes in high-pressure fluids used in spacecraft systems was assigned to an independent consultant (Arthur D. Little, Inc., Cambridge, Massachusetts). Interim reports from this consultant are included in the appendixes; the final report from this consultant will be issued as a separate report at a later date. 2 SECTION A - DESCRIPTION OF TANKS IN USE ON THE APOLLO SPACECRAFT GENERAL DESCRIPTION A description of the Apollo command module (CM), service module (SM), and lunar module (IM) is shown in table A-I. For convenience, the tanks have been grouped by module and use. For example, the CM reaction con­ trol system (RCS) tanks are grouped together and are the first tanks listed. Abbreviations have been used sparingly to facilitate reading. When used, the abbreviations and letters are explained in footnotes at the bottom of the tables. Table A-I lists the engineering data that are necessary for the thermodynamic and kinetic calculations of hypothetical reactions in or surrounding the tanks. These data also give a frame of reference for discussions of compatibility-, contamination, and catalysis. Finally, they give a concise review of the tankage aboard the Apollo spacecraft. SERVICE CONDITIONS The normal service conditions for the CM, SM, and LM tanks are described in table A-II. The data were compiled to permit meaningful calculation of reaction processes in high-pressure systems. Table A-II lists the normal and operating ranges for pressure, temperature, volume or quantity, and flow rates for the tanks to which these parameters are applicable. In those cases where complete information was not available for a given tank, a line is drawn down the column. The operational parameters never exactly match the normal operating parameters; instead, they vary within a small range- When the variance was evenly about a mean value, the mean value is given with plus and minus limits. In other cases, a range is given. The operation and qualification data in table A-II were derived from several sources, among which were the following: telemetry data from sensors aboard Apollo 12 and 13; vendors statement of testing (ref. A-l); pressure-vessel olerating-criteria specifications (refs. A-2 and A-3); and NASA reduced, collated flight data (ref. A-4). :3 PREDICTABLE FAILURE MODES If a tank on the spacecraft system failed, it would do so by one of two brittle fracture failure modes: (1) fragmentation or (2) tearing caused by brittle fracture failure. Brittle fractures occur at strength levels that are belov material-yield strength levels and are caused by preexisting flaws. Material toughness, operating stress level, flaw location, and flaw size influence the characteristics of the brittle fracture. The brittle fracture failure modes for the Apollo tanks are tabulated in table A-111. The failure mode predictions are for preexisting surface flaws, maximum design operating pressures (mop), material properties per specification, and vessel dimensions within design tolerances. Alteration of any of the normal vessel characteristics by physical damage to the tank, overpressure, improper heat treatment, or fabrication errors will change the predicted failure mode. OTHER FAIL-URE MODES Another failure mode is the ductile or overstress failure mode. An overstress condition as high as the ultimate strength of the tank material probably will result in fragmentation of the tank. This failure mode is not predictable by fracture mechanics techniques. Mechanisms are dis- cussed briefly in the following paragraphs for reactions that can occur in the tank that may result in ductile or overstress failure. Ductile failure results from pressure buildup processes inside the .enclosed area. Pressurization can be caused by chemical and physical reactions that occur within the confined fluid. Failure can result from any of several reaction mechanisms. Pressure buildup can result from exothermic or heat-liberating reactions in a combined space. The pres- sure may be increased by the addition of gaseous products resulting from solid or liquid reactants. The pressure also may be increased by an increase of liquid products compared to liquid reactants. The decomposition of hydrazine induced by rust illustrates the above mechanism. The complete decomposition of hydrazine by metallic oxides yields two molecules of water for each hydrazine molecule reacting. " + 2Fe 0 -2H 0 + 2Fe0 + Heat H2-2 2 (2) + (S (2) 3(s) 'qg) Liquid-product insertion into the confined space will increase the pres­ sure. The production of gaseous nitrogen will contribute to the pres­ sure, and the liberation of heat during the reaction will contribute further to pressure buildup. Pressure buildup can occur in some systems because of catalytic + + decomposition of container fluids. Metallic ions, such as Fe.++ Ni +++ r+++ ++ Al Cr or Cu. can be introduced either as impurities or from the vessel walls. This possibility exists with all hydrazine and sub­ stituted hydrazine fuels. The products are a mixture of NH3 , N2 , and H2 In all cases, an excess of product molecules compared to reactant mole­ cules will result, and the container will experience a pressure increase. Under other circumstances, tank surfaces that are inert or passivated may be
Details
-
File Typepdf
-
Upload Time-
-
Content LanguagesEnglish
-
Upload UserAnonymous/Not logged-in
-
File Pages238 Page
-
File Size-