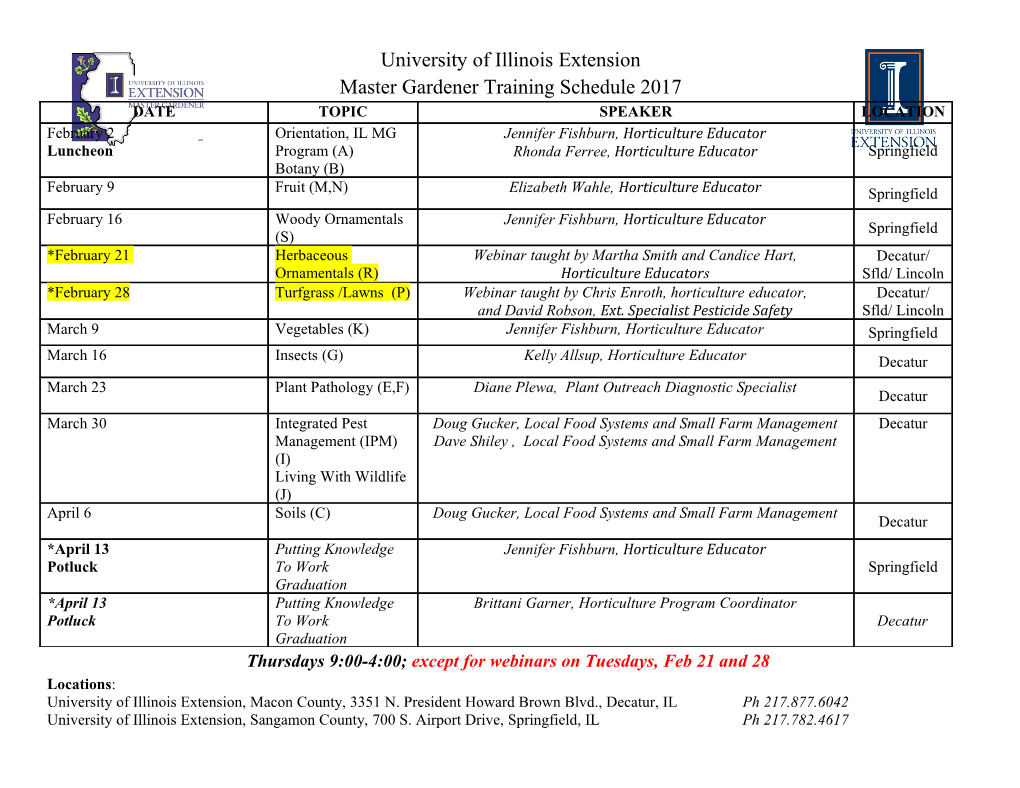
Proc. Nati. Acad. Sci. USA Vol. 90, pp. 1166-1171, February 1993 Colloquium Paper This paper was presented at a colloquium entitled "Molecular Recognition," organized by Ronald Breslow, held September 10 and 11, 1992, at the National Academy of Sciences, Washington, DC. Two light-transducing membrane proteins: Bacteriorhodopsin and the mammalian rhodopsin H. GOBIND KHORANA Departments of Biology and Chemistry, Massachusetts Institute of Technology, 77 Massachusetts Avenue, Cambridge, MA 02139 ABSTRACT Site-directed mutagenesis has provided in- Visual Receptors Bacteriorhodopsin sight into the mechanisms of action of bacteriorhodopsin and rhodopsin. These studies are summarized here. HC CH3 I - CH3 -. 1 : 3 I A * a xix ..A Bacteriorhodopsin was discovered and identified as a light- HO dependent proton pump in the early 1970s (1), whereas the CH3 i- . discovery ofrhodopsin, the dim-light vision photoreceptor, is H.- well over 100 years old (2). The latter serves as an example 11 -cis All-trans par excellance of the superfamily of seven-helix, G protein- coupled receptors. Rhodopsin and the color vision receptors as well as bacteriorhodopsin and related proteins in Halo- bacterium halobium all form a group that uses retinal as the H3C CH3 HH3 n chromophore. The chromophore is invariably linked to the 3li3ran I E-amino group ofa lysine residue as a Schiffbase. The action c..-i s oflight involves specific isomerization ofa double bond in the All-trans I3-c6IS chromophore (Fig. 1) (light transduction). This isomerization couples to specific conformational changes in the proteins FIG. 1. The retinal chromophores and their light-induced (signal transduction). isomerizations in visual receptors and bacteriorhodopsin. formation in the membrane-embedded helices. This was done BACTERIORHODOPSIN by replacing amino acids that could form hydrogen bonds by Electron diffraction work (3, 4) suggested a seven-helix those that could not. Further, the acidic amino acids that structural motif for bacteriorhodopsin. This finding had a could function in protonated/deprotonated states were re- profound impact on our thinking about polytopic integral placed by corresponding neutral amino acids (e.g., Asp -+ membrane proteins that were subsequently discovered. In Asn). In addition, as far as possible, the substituting amino the 1970s and 1980s, the photocycle in bacteriorhodopsin acids were smaller than the corresponding ones in the native received a great deal of attention in order to understand the protein. Table 1 shows the main amino acid replacements that features of proton translocation (5). were made, although a considerable number of additional In our work on bacteriorhodopsin the initial aim was to replacements have since been made. All of these replace- understand its protein chemistry, orientation in the mem- ments were point mutations; i.e., one amino acid was re- brane, delipidation, and reconstitution ofthe proton pumping placed at a time (8-13). function in vesicles. During these studies the remarkable The heterologous expression system in most of the mutant discovery was made that bacteriorhodopsin could be com- work used Escherichia coli, although more recently, efficient pletely denatured under a variety of conditions (e.g., treat- expression of the bacterioopsin gene and mutants has been ment with trifluoroacetic acid) and it would refold essentially developed by transformation in H. halobium (14). Bacterio- quantitatively to native functional structure (6). This finding proved to be critically important in subsequent structure- rhodopsin and mutants do not fold correctly in E. coli; hence function studies. We focused on isolation and characteriza- the mutants are purified (a two-step procedure) in denatured tion of the bacterioopsin gene, developing systems for ex- form and these are then folded and reconstituted by the pression of the gene, site-specific mutagenesis, and, subse- standard procedure (Fig. 3). The experiment is doubly infor- quently, understanding how the light-dependent proton mative. First, it shows the rate of folding of the protein as transport worked. reflected by the formation of the chromophore. These rates Single Amino Acid Replacements in Bacteriorhodopsin. A usually vary widely from that ofthe wild-type opsin. Second, number of physical scientists (M. Polanyi, T. Shedlovsky, J. if there are shifts in the UV/visible spectra from the wild Griffith, L. Onsager, M. Eigen) proposed the idea of an type, these are indicated by the above procedure. extended hydrogen-bonded system for facilitated proton con- C C C duction in ice (Fig. 2). This was extended by Nagle and I Morowitz (7) to proton transfers in proteins. H+ O\ '0\ -O The first aim in our work was to test the above idea in bacteriorhodopsin by interrupting possible hydrogen bond C c C The publication costs of this article were defrayed in part by page charge payment. This article must therefore be hereby marked "advertisement" FIG. 2. Hydrogen-bonded water molecules forming a proton in accordance with 18 U.S.C. §1734 solely to indicate this fact. conductance system. 1166 Downloaded by guest on September 25, 2021 Colloquium Paper: Khorana Proc. Natl. Acad. Sci. USA 90 (1993) 1167 Table 1. Replacements of individual amino acids Table 2. Amino acids whose replacement seriously affects in bacteriorhodopsin proton pumping by bacteriorhodopsin in steady state No. of Mutation H+ pumping, % Wild residues type Substitution(s) None 100 Ser 13 Ala Asp-85 - Ala 0 Thr 18 Ala, Val Asp-85 - Asn 0 Asp 9 Asn, Glu, Ala Asp-85 Glu 39 Glu 9 Asp, Gln, Ala Asp-96% Ala 2 Tyr 11 Phe Asp-% - Asn 3 Pro 11* Leu, Ala, Gly Asp-96 Glu 82 Arg 7 Gln Asp-212 - Ala ND Trp 6 Phe Asp-212 - Asn 15 The amino acids in each set in the wild type were individually Asp-212 - Glu 6 replaced as indicated above (8-13). Arg-82 Ala 30 *Only the three in helices were mutated. Arg-82 - GIn 66 Early efforts at site-specific mutagenesis by oligonucle- ND, not determined. otide-directed mutagenesis were unsuccessful. A highly ef- biophysical methods-e.g., time-resolved spectroscopy in ficient and successful strategy was later developed which was collaboration with different groups [Maarten Heyn (Freie based on the total synthesis of the opsin gene containing University, Berlin), Ken Rothschild (Boston University), unique restriction sites placed at fairly uniform intervals. Vladimir Skulachev (State University of Moscow), and Tim Mutagenesis then involved replacement of DNA fragments Marinetti (Rockefeller University) and their colleagues]. by synthetic counterparts containing the desired codon Thus, Heyn and Skulachev and their coworkers studied the changes. Asp-96 -- Asn change and showed slowing of both the decay All of the mutants containing single amino acid replace- of the M intermediate and the associated proton movement ments folded in lipid/detergent/retinal mixtures and formed (15). They also showed that Asp-96 is the internal proton bacteriorhodopsin-like chromophores. Studies of their spec- donor in the tral properties and proton pumping showed three types of reprotonation of the Schiff base (16). Further, mutants. Type 1 mutants showed no effect on the UV/visible Asp-85, Asp-212, and Arg-82 were shown to affect the proton spectrum or on proton pumping. Type 2 mutants showed release in the early phase of the photocycle (17). Fourier spectral shifts but no marked effect on proton pumping. The transform IR spectroscopy by Rothschild and coworkers (18) spectral shifts, usually to the blue, arise from the fact that the proved valuable in ascertaining protonation/deprotonation amino acids being altered normally interact with the chro- states of the aspartic acids in bacteriorhodopsin. These mophore. About 20 amino acids thus identified are presumed results and those of other groups-e.g., Osterheldt and to line the binding pocket of retinal (6). A similar conclusion coworkers (19, 20)-showed that Asp-85 is the acceptor of has been drawn by Henderson et al. (4) from their structural the proton from the protonated Schiff base in the early phase studies. Type 3 mutants, constituting a small group of amino of the photocycle (Fig. 5). In the second half of the photo- acids, severely affected proton pumping in the steady state cycle, the intermediate M is reprotonated internally by pro- (Table 2). Of these, the mutation Asp-85 -- Asn completely tonated Asp-96. Asp-96 is then reprotonated from the cyto- abolished proton pumping. Thus the main players in proton plasmic side between the N and 0 intermediates (21). transport by bacteriorhodopsin are as shown in Fig. 4. Fig. 6 shows a model of the proton pump which is consis- Replacement of several additional amino acids has also been tent with the present data. shown to affect proton pumping, but further work is neces- The results summarized above are consistent with the sary to assess the significance of these results. established facts that the pump transports H+ ions from the While the above findings provided critical information regarding the amino acids mainly involved in proton transfers A B C D E F G during the photocycle, their specific roles needed to be determined. These were studied in detail by a variety of s AP EpA Inside E R Q24-ST E GA E SD2 GAAEP VF GE D V .-AtA Protein CCE - A VDAD GF R %. 0 0 F FT'R (280nm) KKG FKIF LL V A G LVY F LL F LA L@ - V 'VL --TT!T L LOL L A L R FG.F T L I; V L GVL A ML VT A® o Al 600 1200 1800 T A A no0 Time (sec) LT o / ILP P V M~~~ P Phospholipid - Detergent) G; T G W V F& wL LM AT, G L F 'ATF LW1E L G R G ,a-opoprotein 250 350 450 550 650 750 VpFGU Outside Wavelength (nm) FIG. 4. A secondary structure model of bacteriorhodopsin show- FIG.
Details
-
File Typepdf
-
Upload Time-
-
Content LanguagesEnglish
-
Upload UserAnonymous/Not logged-in
-
File Pages6 Page
-
File Size-