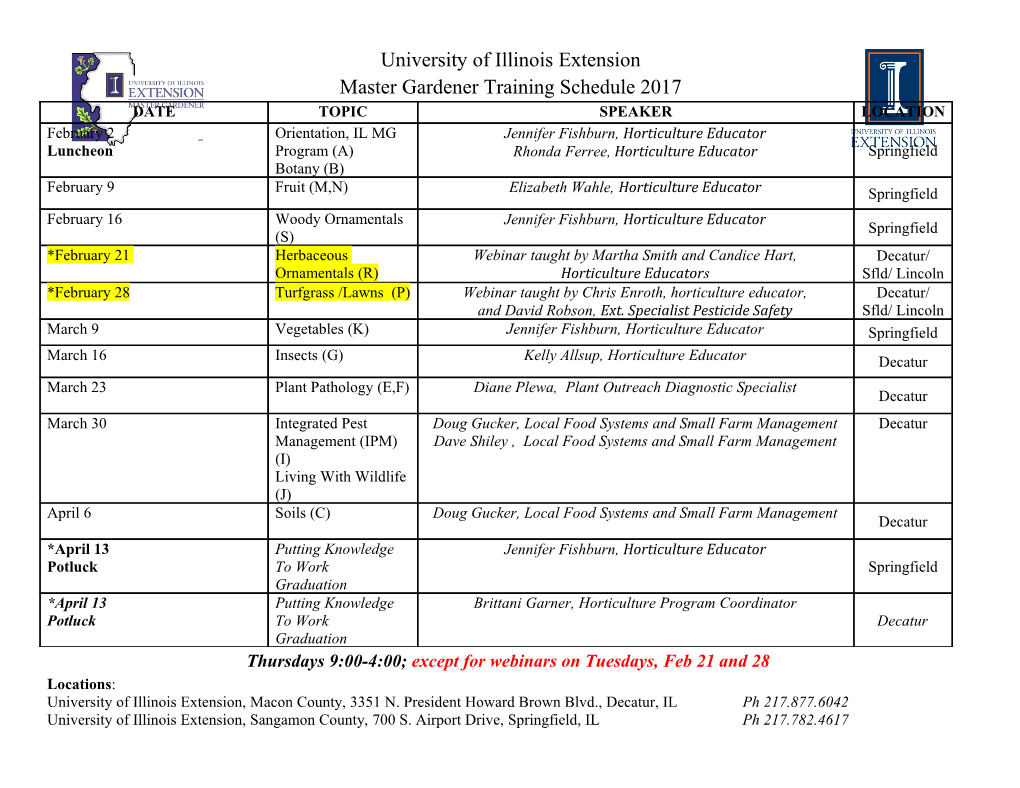
Chapter 13 STRUCTURAL ORGANISATION AND BIOMIMESIS OF NATURE’S POLYMER COMPOSITES Parvez Alam∗ Centre for Functional Materials, Abo Akademi University, Turku, Finland Abstract In nature, there are many examples of complex hierarchical material designs where weak constituent polymers assemble into composites with exceptional mechanical properties. These structures are attracting considerable attention in the scientific and engineering communities as sources of inspiration for polymer composite design. Nat- ural polymers or, biopolymers, may include proteins, polypeptides and polysaccha- rides. These biopolymers are the essential building blocks of hierarchical polymer composites in nature. This chapter elucidates a variety of different polymer composite structures from nature; how they are organised, and the specialised designs that pro- vide for superior functionality. Recurring concepts in biological material structures will also be explained and hence, the chapter will draw upon the elements of structural biology in context to biomimetic and bioinspired composites science. PACS 82.35.Pq, 87.15.rp, 87.85.jf, 87.15.-v, 81.05.Qk Keywords: Biological structures and biomimesis, biopolymers, biomimetics, mechanics, composites, biostructures AMS Subject Classification: 74R10, 74C10, 74L15 1. Introduction 1.1. Bioinspiration A holistic approach to determining the structure-property relationships of biological materials, most recently termed biomateriomics (Cranford and Buehler 2012), is a neces- ∗E-mail address: parvez.alam@abo.fi (Corresponding Author). Parvez Alam, Adjunct Professor of Com- posite Materials and Biostructures, Centre for Functional Materials, Abo Akademi University, 20500, Turku, Finland. Book: New Developments in Polymer Composites Research; Ed. Laske, S. ISBN: 978-1-62948-343-6 − AUTHOR’S PRE-PRINT 2013 326 Parvez Alam sary precursor to biomimetic and bioinspired materials science. By analogy, a robot consist- ing of moving parts, a motor, a sensory system, a power supply and a processor functions only as a robot when all five of these criteria are present. There is no robotic function in any of the individual robotic parts, nor indeed will the robot function should any one of the parts be missing. Similarly, biological structures, or biostructures, when broken down into their constituent elements lack the superior properties they would otherwise exhibit when all the components function in unison as a single composite body. Notably, the majority of biostructures are polymer based composites and as such are dominated by secondary inter- actions. This being the case, it could be easy to assume that biostructures lack the qualities of high stiffness and strength. Quite to the contrary, many biostructures exhibit respectable mechanical properties, and when factored by density, establish their strength–to–weight su- periority over many man–made materials (Chen et al. 2008). It is noted that the range of properties is high in natural materials, i.e. many natural polymer composites will display more modest properties of strength and stiffness; however, this is in itself expected since natural materials are suitably adapted to environmental and intrinsic factors and quite sim- ply, not all these materials need to be mechanically stiff and strong given their application and utility in nature. Numerous natural polymer composites have an outstanding ability to absorb mechanical energy (termed toughness) and will fracture after experiencing consider- able mechanical work. Figs 1 and 2 provide qualitative property maps for natural materials. Fig. 1 also compares the elastic modulus of natural materials to common engineering ma- terials. Figure 1. Elastic modulus/density relationships for engineered and natural materials (adapted from Ashby (1989) and Wegst and Ashby (1994)). Structural Organisation and Biomimesis of Nature’s Polymer Composites 327 Figure 2. Toughness/elastic modulus relationships for a range of natural materials (adapted from Ashby (1989) and Wegst and Ashby (1994)). There are common mechanistic concepts by which certain biostructures are able to de- velop properties of high strength, toughness and fracture resistance. The main ones can be considered as: • Structural hierarchy • Crack retardation • Orientation of composite components • Hydrogen bonded networks • Biological mineralisation • Hard-soft segmentation. Scientists in recent times acknowledge that these factors play a vital role in turning ini- tially weak monomeric or polymeric building blocks into mechanically enhanced compos- ite systems. As a consequence, a concerted worldwide effort is being undertaken towards mimicking, or taking benefit from such biostructures. This effort is collectively termed 328 Parvez Alam biomimesis, biomimetics or more generically, bioinspiration. Biomimetics can be subdi- vided into four interconncted stages. 1. Exploration and discovery 2. Understanding behind the science and function of a specific feature (or quality) in the natural organism under scrutiny 3. Mimicry of this feature, or the processes by which it develops, in man-made materials and structures 4. Application of the mimicked feature or process by identifying a niche area in sci- ence/technology where this feature could serve a distinct purpose. Typical fields where biomimetics is currently applied include; robotics, artificial intel- ligence, aerodynamics, fluids and surfaces, smart systems and materials, biomedical appli- cations (including molecular biomimetics) and in engineering composites. 1.2. The Building Blocks of Biostructures Biopolymers and mineral compounds are the two main classes of material that typically conjoin to form composite biostructures. Both classes of material may exist as crystalline, semi crystalline or amorphous subunits that ultimately determine the material properties of the composite biostructure. Moreover, mineral compounds may form meta-stable poly- morphs that can convert to a stable polymorph under certain conditions of pressure and temperature. The following sections describe these essential building blocks of composite biostructures. 1.2.1. Amino Acids Amino acids are named as such because they contain a weak (carboxylic) acid group [-COOH] and an amine group [-NH2]. They are absorbed by biomineralising organisms (e.g. corals), adsorbed to nucleating crystallites during the process of biomineralisation, involved in numerous metabolic processes and can conjoin to form long chain polymers (e.g. proteins). Each amino acid subtly differs with respect to their particular side chain. Resultantly, the molecular lengths, conformations and chemical behaviours of amino acids vary considerably. Figs. 3–6 show the chemical structures for twenty proteinogenic amino acids. Proteins are formed when amino acids form polypeptide chains. Amide bonds are formed when the carboxyl and amine groups from two amino acids react with one another in a condensation polymerisation reaction. A reaction as such, between two amino acid molecules releases one molecule of water. Adjacent amino acids (or proteins) may inter- act through electrostatic and van der Waals forces, the strength of which depends on their molecular conformations, their proximal distances and the types of side chains available for interaction. Structural biopolymers may be built up of polypeptide chains, polysaccharides or a combination of the two. Biopolymers such as collagen, silk, and keratin are built up of Structural Organisation and Biomimesis of Nature’s Polymer Composites 329 Figure 3. Proteinogenic amino acids with hydrophobic side chains. polypeptides, while celluloses and hemicelluloses are constructed from polysaccharides. Chitin is the anomaly in that it is formed of both polypeptide and polysaccharide chains. Celluloses and chitins are the two most abundant biopolymers on earth. While cellulose is most commonly found within the plant kingdom, chitin is a common structural component in the insect kingdom and in the materials of marine organisms. The following sections introduce these two ubiquitous biopolymers and a list of polypeptide based structural pro- teins. 1.2.2. Chitin Chitin has the chemical formula (C8H13O5)n, and its structure is shown in Fig. 7. It is the second most common biopolymer in nature after cellulose. Chitin is a long chain deriva- tive of glucose and has repeat units of N–acetylglucosamine. It is found in the exoskeletons of arthropods such as insects and crustaceans, and in the cell walls of fungi. Chitin is solu- ble in aqueous solutions of some acids and chitin chains tend to pack into nanofibrils with a diameter of ca. 2.5 nm. The nanofibrils connect into larger units known as microfibrils which have a diameter ca. 10 fold higher than single nanofibrils. Chitin forms strong but flexible crystalline structures since there are numerous hydrogen bonding units. The hydro- gen bonds are not strong individually 5–20 kcalmol−1, but when there are many of them they stabilise the network and have a higher possibility of reforming once broken. There 330 Parvez Alam Figure 4. Proteinogenic amino acids with electrically charged side chains. are three forms of chitin in nature; α–chitin, which is the most abundant structure consists of both parallel and anti-parallel molecular strands packed tightly in an orthorhombic unit cell, β–chitin, which exists in parallel chains packed in a monoclinic unit cell, and γ–chitin, which is rarely found in nature and is regarded an intermediate crystalline form of α–chitin and β–chitin. 1.2.3. Cellulose Cellulose is the
Details
-
File Typepdf
-
Upload Time-
-
Content LanguagesEnglish
-
Upload UserAnonymous/Not logged-in
-
File Pages59 Page
-
File Size-