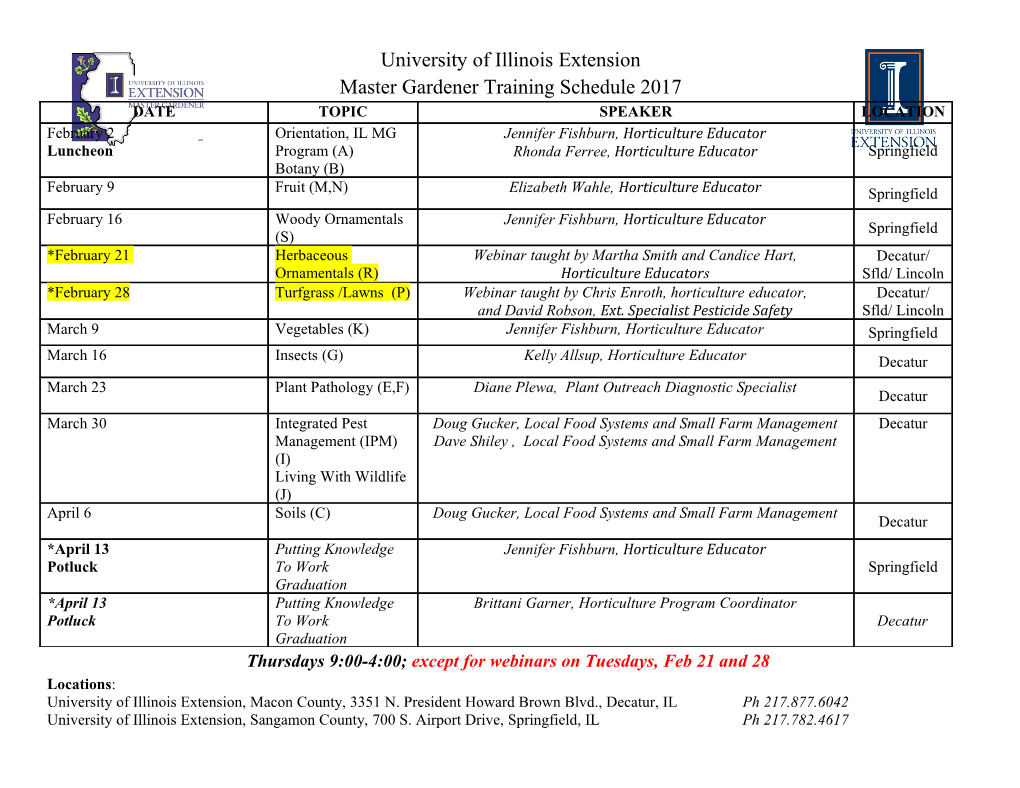
Wear 262 (2007) 684–692 A tribo-testing method for high performance cold forging lubricants Gracious Ngaile a,∗, Hiroyuki Saiki b, Liqun Ruan b, Yasuo Marumo b a Department of Mechanical and Aerospace Engineering, North Carolina State University, Campus Box 7910, Raleigh, NC, USA b Department of Mechanical Engineering and Materials Science, Kumamoto University, 2-39-2, Kurokami 860-8555, Japan Received 31 December 2005; received in revised form 2 August 2006; accepted 3 August 2006 Available online 15 September 2006 Abstract A tribo-testing method based on inducing different deformation patterns at the tool–workpiece interface developed by the authors was used in rating the performance of high quality lubricants. Dies which can induce different levels of maximum surface expansion under localized rod drawing set up were used. The maximum local surface expansion induced ranged from 20 to 500%. The basic feature for this test lies under the assumption that the surface expansion is proportional to the lubricant thinning and breakdown at the tool–workpiece interface. The experimental set up is coupled with die heating facilities used to raise the temperature at the interface so that the influence of temperature on the performance of the lubricant is studied. The performance of several coating-based lubricants was studies under this method. One of the goals of screening the lubricant was to identify possible lubricant candidates for replacing zinc phosphate coating based lubricant for medium forging processes. The results have demonstrated that, the effectiveness of the lubricants varies considerably with changes in the maximum local surface expansion induced at the interface and the change in the interface temperature. Of the six lubricants studied, two lubricants based on calcium and sodium soap were found to be at the same performance level as the conventional zinc phosphate coating + metal soap. © 2006 Elsevier B.V. All rights reserved. Keywords: Tribo-testing; Lubricants; Cold forging; Surface evolution 1. Introduction total failure of the tribo-test. In other words, the data obtained from the tribo-test may not be reliable particularly, if the defor- Lubricants play a key role in cold forging as they reduce the mation modes induced by the test have not been thoroughly high frictional forces occurring at the tool–workpiece interface. studied. Improved lubrication systems are needed, however, as they will In screening high performance lubricants for cold forging of help make net-shape production possible and affordable. The complex components, more careful attention is needed. Most of development of new lubrication systems needs to go hand in these lubricants have good lubricity and it is not easy to distin- hand with the development of laboratory tests. A number of guish which lubricant is superior to the other, unless a tribo-test tests for cold forging processes have been developed to date. employed is sensitive enough to simulate the right deformation Critical reviews on these tests are given by Schey [1], Bay and modes relevant to the specific forging process. An important Hansen [2], and Kawai et al. [3]. The problem pertaining to some aspect for a lubricant meant to be used in complex forging oper- of the tribo-tests developed to date is the failure to reproduce ation is the level of adhesive strength of the lubricant to the similar conditions to those occurring in the actual production parent material and the ability of the lubricant to follow surface environment. The failure of the tribo-tests can be caused by extension without breaking down [4,5]. the deviation of the test characteristics from the actual plant tri- The aim of this paper is to present on the performance of sev- bosystem characteristics. Furthermore, the deviation of variables eral lubricants tested using the tribo-test method based on induc- such as interface pressure, sliding velocity, interface tempera- ing different deformation modes at the tool–workpiece interface. ture, contact time, surface expansion, and the like can lead to a In this method, tools of different geometrical profiles which can induce different levels of maximum local surface expansion are used. The basic feature for this test lies under the assumption that ∗ Corresponding author. Tel.: +1 919 515 5222; fax: +1 919 515 7968. the surface expansion is proportional to the lubricant thinning E-mail address: gracious [email protected] (G. Ngaile). and breakdown at the tool–workpiece interface. 0043-1648/$ – see front matter © 2006 Elsevier B.V. All rights reserved. doi:10.1016/j.wear.2006.08.009 G. Ngaile et al. / Wear 262 (2007) 684–692 685 Fig. 1. (a–c) Localized rod drawing experimental set up. 2. Methodology in Fig. 1b. P = . ε − . ε − . ε The test is modeled such that, tools of different geometrical v 0 0059 1 0 0181 2 0 0528 3 (2) profiles are employed in order to induce different deformation Pc =−0.0601ε1 − 0.0467ε2 − 0.0017ε3 (3) patterns at the tool–workpiece interface under localized rod drawing setup. The tools employed vary from flat, sinusoidal, where ε1, ε2 and ε3 are output strains in mV from the load cell. saw tooth, and multi-surface profiles as shall be discussed in Sec- These expressions were obtained after conducting calibration of tion 2.3. The drawing force is supplied by a 500 kN Hydraulic the load cell. The load cell was designed such that Pc and Pv are cylinder and the die closing force being supplied by 200 kN linear function of strains ε1, ε2 and ε3 shown in Eqs. (4) and (5) hydraulic cylinder. Fig. 1 shows the experimental test set up. [Fig. 1b and c]. The tooling can accommodate round rods of 1000 mm length P = a ε + a ε + a ε and 20 mm diameter. v 1 1 2 2 3 3 (4) Pc = a4ε1 + a5ε2 + a6ε3 (5) 2.1. Determination of interface friction To determine the values for the constants a1, a2, a3, a4, a5, The friction coefficient developed at the tool–workpiece and a6, Eqs. (4) and (5) were arranged to form a function f and interface under this test is given by the following formula. this function was minimized as shown in Eqs. (6) and (7). 1 Pv f = a ε + a ε + a ε − P 2 μ = × tan arctan − φ (1) ( 1 1 2 2 3 3 c) C Pc 2 + (a4ε1 + a5ε2 + a6ε3 − Pv) (6) where, Pv is half the drawing force, Pc the die closing force and φ is the half die angle. C is a correction factor for non-flat dies ∂ 2 (Wavy dies) obtained by dividing the contact area of a non-flat (a1ε1 + a2ε2 + a3ε3 − Pc) die to that of a flat die of the same width. For flat dies C =1. ∂ai The Pv and Pc are determined from Eqs. (2) and (3). The free 2 + (a4ε1 + a5ε2 + a6ε3 − Pv) = 0 (7) body diagram showing the orientation of Pv and Pc can be seen 686 G. Ngaile et al. / Wear 262 (2007) 684–692 Fig. 2. Wavy die geometries. where subscripts i = 1, 2, 3, 4, 5 and 6. Calibration loads for Pc employs dies with surface patterns ranging from flat, sinusoidal, and Pv and their corresponding output strains were substituted saw-tooth, to multi-surface ones. The major die geometrical vari- in the above equations to solve for the constants. ables are contour radii, pitch, root depth and the angle subtending the ridges. To determine the maximum local surface expansion 2.2. Tool–workpiece interface temperature induced by these dies, finite element (FE) simulation is used. Surface expansion values are highly dependent on deformation In order to emulate realistic forging temperatures the setup is modes, part complexity and the material properties, particularly, designed such that the dies are electrically heated to a controlled the strain hardening index [8,9]. Fig. 2 shows examples of wavy temperature. This is to embody similar thermal characteristics dies. at the tool–workpiece interface as those occurring in an actual cold forging plant where the temperature normally reaches val- 2.3.1. FE modeling ues of about 200 ◦C [6,7]. As shown in Fig. 1c, the die holder is 2D FE simulations were carried out to study the local sur- designed with provisions for inserting heating coils. The desired face expansion as the dies pinch the material to a predetermined tool–workpiece interface temperature which can be varied from reduction. The simulations were carried out using the commer- room temperature to 300 ◦C is controlled by a control panel cial FE software DEFORM. The FE simulations emulate the first [Fig. 1a]. The load cell is provided with water jackets [Fig. 1c] stage during the localized rod drawing process. FE simulation where water is circulated to restrict the excessive heat transfer for the second stage, i.e., drawing was not carried out. There- to the load cell. By controlling the volume flow rate of water fore, the contribution of drawing to the surface expansion is not in the cooling system, the temperature distribution at the load reported here. The FE simulations were conducted for Flat dies, cell structure is kept below 25 ◦C. The major reasons for incor- Wavy-2 and Wavy-3 dies. For all the simulations a 12 mm diam- porating the cooling system is to prevent any variation of stress eter rod was pinched by advancing the dies from each side by due to temperature increase at the columns where strains are 2 mm. The flow stress for the specimen was σ = 800εn N/mm2. measured and also suppress any change in strain gauge char- A quarter FE model with 3000 elements and a friction factor of acteristics which might be caused by the thermal loading.
Details
-
File Typepdf
-
Upload Time-
-
Content LanguagesEnglish
-
Upload UserAnonymous/Not logged-in
-
File Pages9 Page
-
File Size-