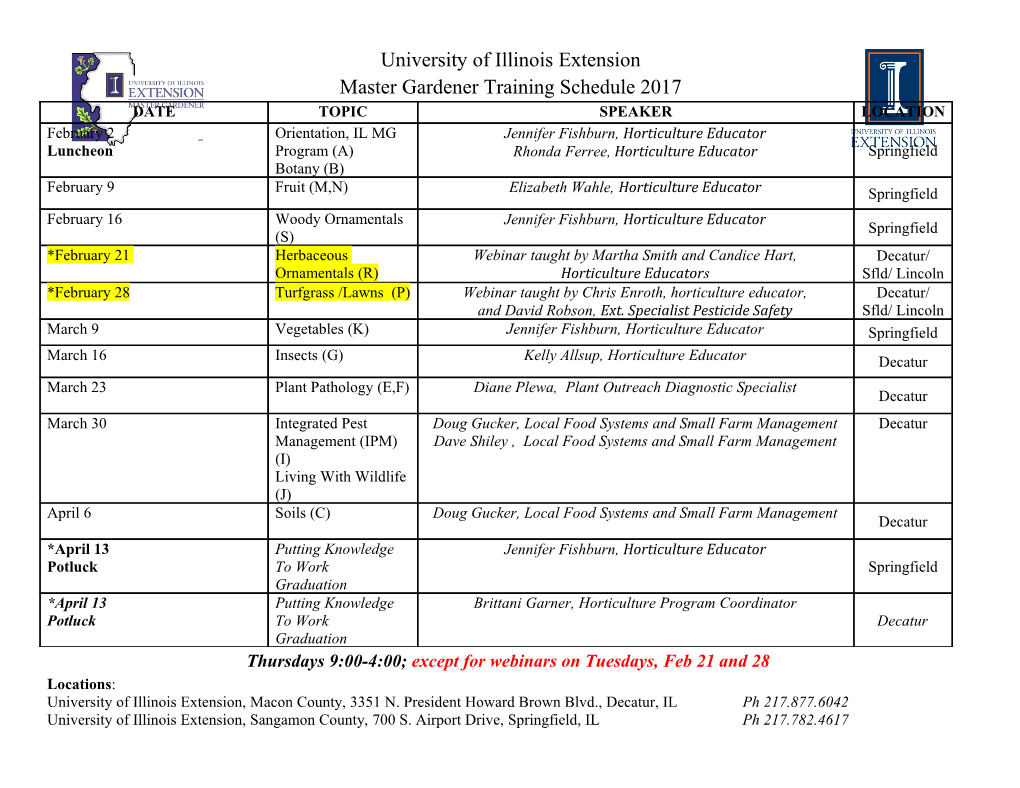
Revisiting the structures of several antibiotics bound to the bacterial ribosome David Bulkleya,1, C. Axel Innisb,1, Gregor Blahab, and Thomas A. Steitza,b,c,2 aDepartments of Chemistry and bMolecular Biophysics and Biochemistry, Yale University, cHoward Hughes Medical Institute, New Haven, CT 06511 Edited* by V. Ramakrishnan, Medical Research Council, Cambridge, United Kingdom, and approved August 17, 2010 (received for review June 18, 2010) The increasing prevalence of antibiotic-resistant pathogens rein- decided to revisit the structure of chloramphenicol bound to the forces the need for structures of antibiotic-ribosome complexes ribosome for several reasons: (i) only one such structure is avail- that are accurate enough to enable the rational design of novel able, and it originated from the same study that yielded the struc- ribosome-targeting therapeutics. Structures of many antibiotics ture of a bacterial ribosome in complex with erythromycin that in complex with both archaeal and eubacterial ribosomes have was inconsistent with later structural work at higher resolution; been determined, yet discrepancies between several of these (ii) in contrast with the distinctive lactone ring of macrolides, models have raised the question of whether these differences arise chloramphenicol lacks obvious structural features that would from species-specific variations or from experimental problems. facilitate its placement into a medium resolution electron density Our structure of chloramphenicol in complex with the 70S ribo- map; (iii) a number of minor inconsistencies between the sole some from Thermus thermophilus suggests a model for chloram- available structural model and biochemical data warrant addi- phenicol bound to the large subunit of the bacterial ribosome tional validation of the former (14–17). The structures of the that is radically different from the prevailing model. Further, our erythromycin, azithromycin, telithromycin, and chloramphenicol structures of the macrolide antibiotics erythromycin and azithro- complexes with the T. thermophilus 70S ribosome reported here mycin in complex with a bacterial ribosome are indistinguishable are all inconsistent with the earlier published structural studies from those determined of complexes with the 50S subunit of with the D. radiodurans large subunit, but the macrolide com- Haloarcula marismortui, but differ significantly from the models plexes are in complete agreement with the structural conclusions that have been published for 50S subunit complexes of the eubac- from the published H. marismortui large subunit complexes. terium Deinococcus radiodurans. Our structure of the antibiotic Results telithromycin bound to the T. thermophilus ribosome reveals a lactone ring with a conformation similar to that observed in the Datasets for the chloramphenicol, erythromycin, and azithromy- H. marismortui and D. radiodurans complexes. However, the cin ribosome complexes were collected from single crystals, alkyl-aryl moiety is oriented differently in all three organisms, and whereas the data from the complex with telithromycin was the contacts observed with the T. thermophilus ribosome are con- obtained from three separate crystals. All crystals belonged to the 2 2 2 sistent with biochemical studies performed on the Escherichia coli primitive orthorhombic space group P 1 1 1 and diffracted to – ribosome. Thus, our results support a mode of macrolide binding 3.1 3.3 Å. Statistics for the data can be seen in Table S1. that is largely conserved across species, suggesting that the quality Chloramphenicol. and interpretation of electron density, rather than species specifi- Chloramphenicol binds directly to the A-site city, may be responsible for many of the discrepancies between the crevice on the 50S ribosomal subunit, occupying the same loca- models. tion as the aminoacyl moiety of an A-site tRNA (Fig. 1) (18, 19). The placement of this drug in the immediate vicinity of the in- crystal structure ∣ structure-based drug design coming A-site tRNA is consistent with biochemical experiments (20, 21). The location and orientation of chloramphenicol that we observe in our complex is completely different from the model ntibiotics that target the translational machinery of bacterial that has been published for the D. radiodurans 50S subunit com- cells are potent inhibitors of prokaryotic pathogens (1), but A plex (11) (Fig. 1 A–C). Superimposition of the ribosomal RNA increasing rates of antibiotic resistance among bacteria often from the two structures shows that the two chloramphenicol dampen the effectiveness of common, clinically used antibiotics models are related by a rotation of approximately 180° and that (2–5). Recent work has shown that structure-based drug design the planes of the benzyl rings are orthogonal to one another. exhibits the potential to overcome this problem by chemically Additionally, the ion(s) anchoring the molecule to the ribosome linking together pairs of known classes of ribosomal inhibitors differ in their location, number, and identity; the D. radiodurans to generate novel antibiotics (6–8). The wealth of ribosomal anti- model posits two putative and as yet unproven magnesium ions biotics and corresponding resistance mutations suggests that many additional chemical derivatives of existing antibiotics might be created and could be useful in dealing with resistance, but con- Author contributions: D.B., C.A.I., G.B., and T.A.S. designed research; D.B., C.A.I., and G.B. flicting crystallographically determined models for how some of performed research; D.B., C.A.I., and G.B. analyzed data; and D.B., C.A.I., and T.A.S. wrote these antibiotics are bound and oriented could be an impediment the paper. to the design of new hybrid molecules. For example, structures Conflict of interest statement: Thomas A. Steitz is a scientific advisor for Rib-X Pharmaceuticals, a product-driven small molecule drug discovery and development of several macrolide and ketolide antibiotics in complex with company focused on the structure-based design of unique classes of antibiotics. Deinococcus radiodurans the large ribosomal subunits of and *This Direct Submission article had a prearranged editor. Haloarcula marismortui (9–12) have shown significant differences Freely available online through the PNAS open access option. in the orientations and conformations of the drugs, in particular Data deposition: The atomic coordinates for the various ribosome-antibiotic complexes with respect to the lactone rings and the contacts they make with have been deposited in the Protein Data Bank, www.pdb.org [PDB ID codes 3OGE/3OGY/ the ribosome. Because it has been suggested that the discrepan- 3OH5/3OH7 (chloramphenicol), 3OHC/3OHD/3OHJ/3OHK (erythromycin), 3OHY/3OHZ/ cies between these models result from species-specific differences 3OI0/3OI1 (azithromycin), and 3OI2/3OI3/3OI4/3OI5 (telithromycin)]. in the ribosomal RNA (13), we chose to address these issues by 1D.B. and C.A.I. contributed equally to this work. determining the structures of the 70S ribosome from the eubac- 2To whom correspondence should be addressed. E-mail: [email protected]. Thermus thermophilus terium in complex with the antibiotics This article contains supporting information online at www.pnas.org/lookup/suppl/ erythromycin, azithromycin, and telithromycin. In addition, we doi:10.1073/pnas.1008685107/-/DCSupplemental. 17158 of 17163 ∣ PNAS Early Edition www.pnas.org/cgi/doi/10.1073/pnas.1008685107 Downloaded by guest on October 2, 2021 Fig. 1. Interaction between chloramphenicol and the ribosome. (A) An unbiased 3.2-Å-resolution Fo-Fc difference electron density map contoured at þ3σ calculated using amplitudes from T.th. 70S ribosome crystals soaked with 500 μM chloramphenicol. The chloramphenicol moiety is shown in orange, along with a potassium ion mediating the interaction between the drug and the ribosome. (B) The same electron density as shown in A, with the chloramphenicol moiety and two magnesium ions modeled in the D.ra. 50S structure (11) shown in red. The T.th. 70S ribosome and the D.ra. 50S subunit structures were superimposed using equivalent phosphate atoms in the 23S rRNA and the program Lsqman (54). The superimposed structures had an rmsd of 1.21 Å for 2,800 atoms. (C) Overlay of the chloramphenicol molecules modeled in the T.th. 70S (orange) and D.ra. 50S (red) complex structures. Both the model coordinates and the view are the same as in A and B.(D) Overlay of the chloramphenicol molecule modeled in the T.th. 70S complex (orange) and the anisomycin molecule modeled in H.ma. 50S complex (blue). The superimposed structures had an rmsd of 1.53 Å for 2,800 atoms. (E) Interactions between chloramphenicol and the T.th. 70S ribosome. The drug is shown as green sticks, and key interacting residues in the ribosome are shown in white. The potassium ion is displayed as a gray BIOPHYSICS AND sphere. (F) A surface representation of the binding pocket for chloramphenicol in the T.th. 70S ribosome, showing surface complementarity between the drug COMPUTATIONAL BIOLOGY (orange) and the A-site crevice in the ribosome as well as the aminoacyl group (blue) of bound phe-tRNA (37). holding the drug in place, whereas our data implicate a single col is bound, which is consistent with the structure we observe potassium ion whose location in the peptidyl transferase center (29, 30) (Fig. S1D). (PTC) is consistent with previous biochemical and crystallo- The acetylation of chloramphenicol at the methylene hydroxyl graphic experiments
Details
-
File Typepdf
-
Upload Time-
-
Content LanguagesEnglish
-
Upload UserAnonymous/Not logged-in
-
File Pages6 Page
-
File Size-